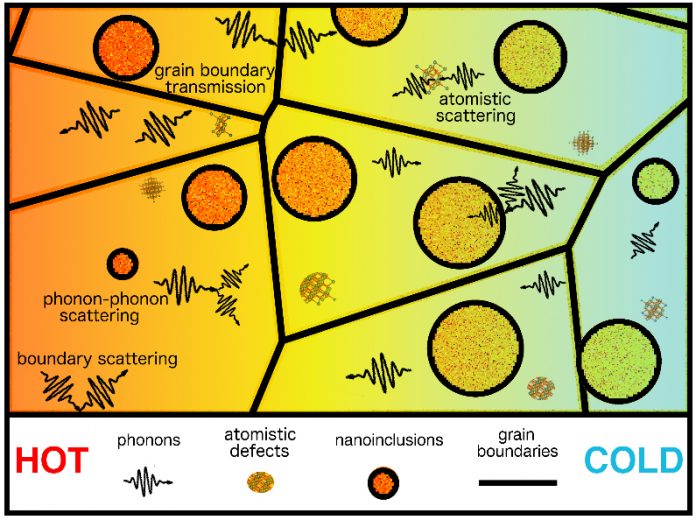
Professor Neophytos Neophytou sheds some light on nanostructures and explains how the Horizon 2020 NANOthermMA project could eventually enable a strong boost in thermoelectric technology
Heat is a by-product of all our energy conversion processes taking place anytime useful work is performed and is abundant all around us. It can be found in different scales. Large quantities of heat are encountered in most industrial processes, think for example, the steel industry and power stations. Medium-level waste heat is encountered in the exhausts and alternators of cars, for example, where almost two-thirds of the fuel is wasted. A good example of micro-scale heat is what our bodies provide.
In the urgent search for green energy technologies, it is tempting to find ways to harvest these large quantities of heat and convert them into useful electrical power, leading to the reduction in the use of fossil fuels. The process of converting waste heat into electricity is not new. It dates back to the 1800s and the work of Alessandro Volta and Thomas Seebeck, with the latter giving his name to the Seebeck effect. Namely, when a special type of material is subject to a temperature gradient, an electrical voltage is created across it. These are called thermoelectric materials.
The problem with thermoelectrics is their low efficiency, which is quantified by the so-called ZT figure of merit. The best materials traditionally exhibit ZT of around unity, which corresponds to approximately 10% of the Carnot efficiency. It is estimated that a ZT around three is needed to enable large-scale applicability of this technology. State-of-the-art materials are alloys and compounds of bismuth, tellurium, and lead, which presents another problem, as these are extremely rare and/or toxic. Thus, to-date, thermoelectric materials have only found use in niche applications such as deep space missions.
In a simplified manner, an efficient thermoelectric process requires the material that is placed between the hot and cold ends of the temperature gradient to possess the following properties:
- High electrical conductivity, such as the electrons that absorb the heat at the hot side propagate easily to the cold side.
- Electrons from the cold side should be stopped from propagating and counteract the hot electron flux. The ability of a material to separate these two fluxes is essentially the Seebeck coefficient.
- The material should have very low thermal conductivity. In this way, the heat energy is transferred to the electrons, rather than propagate through the material in the form of phonons.
Nanostructures can effectively slow the flow of phonons and reduce the thermal conductivity drastically (see Fig. 1), without reducing the electrical conductivity. Because of this, in the last decade, nanostructuring became state-of-the-art in thermoelectrics, with ZT values of two being demonstrated for many materials. It has been so successful, that it has already reached its limits in terms of lowering the thermal conductivity. Further improvements will need to come from the electrical conductivity and the Seebeck coefficient. The problem that arises, however, is that these two quantities are inversely proportional to each other, and any efforts to increase one, degrade the other.
NANOthermMA ERC StG
This is where the NANOthermMA project comes, with the goal to theoretically identify nanostructured material designs that relax the adverse interdependence of these two parameters. The project has shown that, in contrast to pristine materials for which the electrical conductivity and Seebeck coefficient are inversely proportional to each other, this does not strictly apply for nanostructured materials. Through a series of numerically intensive simulations using advanced software developed within the project, NANOthermMA has demonstrated theoretically how engineering at the nanoscale can break the adverse interdependence of these parameters and even achieve a 20-fold increase in the power factor – which is the product of the electrical conductivity and Seebeck coefficient squared, a quantity that directly affects the ZT.
For this to be achieved, the project has developed a ‘recipe’ whose main ingredient is the formation of nanodomains of specified sizes separated by boundaries, e.g. nanograins and grain boundaries as shown in Fig. 1. Within this geometry an additional species of dopant atoms is inserted at specified regions – dopant atoms are impurities which increase the electrical conductivity of the material. By carefully designing the sizes of the domains, the nature of the boundaries and the distribution of the dopants, what can be achieved is for the electrical conductivity to be primarily determined by the nanodomains whereas the Seebeck coefficient to be determined by the boundaries, and the two can be tuned independently.
The proposed ‘recipe’ is independent of the choice of the material used, and the project investigates the design details for many material candidates to identify the highest performers. The design can be further improved by utilising hierarchical nanostructuring. Here, nanoinlusions are inserted within the nanodomains to slow down the heat flow and reduce thermal conductivity even further, but can also independently improve the Seebeck coefficient.
Due to the complexity of electrical and thermal transport in these hierarchically nanostructured materials, advanced theory and simulation tools are needed to guide the design and optimisation process. The project develops such advanced simulators based on analytical methods, semi-classical methods, but also purely quantum-mechanical methods. The former two provide computational ease and simulation flexibility. The latter is computationally more expensive but includes important nanoscale phenomena which are not captured by semi-classical methods.
The challenge now is whether these findings can be realised experimentally. The project has an experimental part whose purpose is to validate the ‘recipe ingredients’ identified. It is expected that confirmation results will be reached towards the last year of the project. If these are realised even partially, it would open the path towards reaching ZT values beyond four and enable a strong boost in thermoelectric technology.
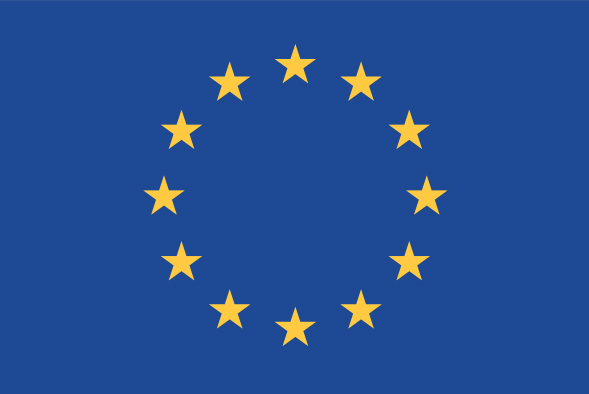
Project name: NANOthermMA
Please note: This is a commercial profile