Prof Dr Stephanie Wehner, Director of the European Quantum Internet Alliance, explains how quantum network technology and a quantum internet could revolutionise communication and connectivity
The internet, an intricate network connecting devices across the globe with classical communication, has profoundly shaped our world. We now stand on the brink of a new type of internet. Imagine an evolution of the internet, one that intertwines the principles of quantum mechanics with our existing digital technology. This is the vision of a quantum internet – an innovation that promises to redefine our understanding of communication and connectivity.
Working in tandem with our traditional ‘classical’ internet, a quantum internet would connect quantum devices globally. Such a network would unlock capabilities that are fundamentally unattainable through classical communication alone. Take, for instance, Quantum Key Distribution (QKD), a striking application of quantum communication. QKD allows two distant nodes to create an encryption key guarded by the immutable principles of quantum mechanics.
This allows secret communication that is future-proof; that is, it is secure even against an eavesdropper equipped with a large-scale quantum computer now or in the future. In a world where data security is paramount, this quantum advantage could be greatly beneficial and is now commercially available in metropolitan areas.
Beyond secure communication, however, we already know an array of transformative applications that highlight the potential of quantum network technology. The potential is immense, and the vision of a quantum internet is to build a universal quantum network that can be programmed to run any type of future quantum network application.
What is a quantum network?
At the heart of these applications lie quantum bits, or ‘qubits’. Unlike classical bits that exist as either ‘0’ or ‘1’, qubits can simultaneously inhabit a state of ‘0’ and ‘1’. Intriguingly, it is impossible to copy arbitrary qubits. Any attempt at duplication can be detected, making them an ideal tool for secure communication. Two qubits can also be entangled, where entanglement forms an inherently private connection that cannot be shared with anything else.
A quantum network allows the transmission of qubits or, more generally, the creation of entanglement between nodes in the network (Figure 1). Such nodes may be simple photonic devices that only allow measuring one qubit at a time or more sophisticated devices.
Unlike in quantum computing, where one can only draw real-world value once one has built a quantum computer that can outperform classical (super-)computers, the path towards bringing benefit to users is more gradual in the domain of quantum networking. Simple photonic devices can already unlock applications such as quantum secure communication in metropolitan areas.
Today, quantum communication is commercially available in metropolitan areas (short distances of up to 100km in fiber) when restricted to simple use cases enabled by QKD. No long-distance quantum networks are deployed today that enable end-to-end quantum communication and, thus, end-to-end quantum secure communications.
Ongoing R&D efforts worldwide work to advance quantum networking in three directions: (1) distance – to connect users in different metropolitan areas and beyond using end-to-end quantum communication; (2) functionality – to enable applications beyond secure communication; and (3) accessibility – to create cheaper devices. Global reach may eventually be attained using a combination of quantum repeaters in fiber networks on the ground, and quantum satellites.
Both are the subject of ongoing R&D efforts. Stages of functionality (Figure 2) have been identified for the development of a quantum internet, where each stage unlocks a larger class of possible user applications. (1)
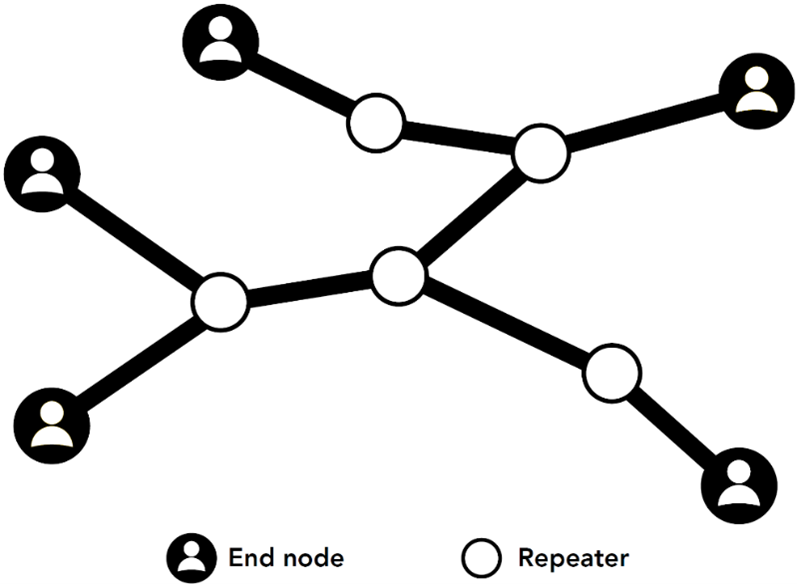
What can one do with quantum network technology?
Using the stages of functionality as a guideline, let us briefly provide more information about applications and potential use cases of quantum network technology.
Prepare and measure stage
This stage contains QKD, which addresses the critical challenge of securing communication in transit and using keys to authenticate access.
It is interesting to note that many devices that can do QKD could, in principle, also be used to provide an advantage in other security-sensitive domains, including, for example, password identification or privacy-preserving analytics.
Entanglement generation stage
This stage unlocks versions of the aforementioned use cases in security with the additional guarantee that the quantum devices are not trusted – a feature known as device independence in quantum cryptography.
Moreover, this stage enables all use cases that exploit the fact that entanglement allows for stronger correlations (4) when measuring the qubit than is allowed classically. It has been shown that practical applications exist for this, enabling remote Bridge players to gain an advantage. (3)
On a more speculative note, it may be interesting to explore whether pre-shared entanglement generated using a quantum network can enhance efficiency in other tasks that require coordination, such as high-frequency trading.
Quantum memory stage
This stage can be reached if the devices connected to the quantum network are quantum processors, that is, quantum computers capable of storing and manipulating a few qubits. Examples of possible use cases in this stage include secure quantum computing in the cloud. (2)
To highlight the breadth of potential use cases, we also remark that a Quantum internet can combine remote sensors for higher-resolution imaging. (5) This has potential applications in astronomy, obtaining sharper celestial images, geological exploration, and identifying potential materials in the ground.
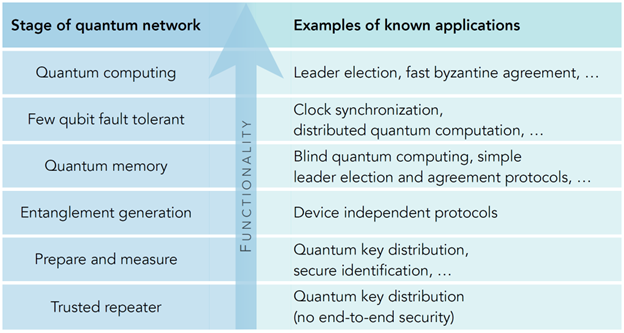
Few qubit fault tolerant stage
This stage is distinguished from the last one in that the quality of the qubits in the quantum processor is very high – specifically, their quality is protected by fault-tolerant quantum computing.
For example, the Quantum internet could reduce communication requirements for solving specific tasks. (6) This could have the potential to enable, for example, faster appointment scheduling across multiple calendars, comparisons of data stored at different network sites, or faster image processing in image recognition tasks. Other examples in this stage include enabling proofs of data deletion. (7)
Quantum internet alliance
The Quantum Internet Alliance (QIA) is a partnership of presently 40 members, including leading actors from academia, and industry in Europe, to build a prototype Quantum Internet. This prototype network will connect two metropolitan area networks via a long-distance backbone.
QIA also provides a platform for Quantum Internet Innovation with opportunities to connect.
References
- Science, 362 (6412), 2018
- NPJ Quantum Information, 3(1), 2016
- Phys. Rev. X 4, 021047, 2014
- Rev. Mod. Phys. 86, 419 (2014)
- Phys. Rev. Lett. 109, 070503, 2012
- Rev. Mod. Phys. 82, 66, 2010
- IEEE ISIT 2019, https://doi.org/10.1109/ISIT.2019.8849661

This work is licensed under Creative Commons Attribution-NonCommercial-NoDerivatives 4.0 International.