Filip Johnsson, Lisa Göransson and Alla Toktarova, explain why decarbonising the steel industry gives us reasons to be cheerful
In Europe, the steel, cement, chemical, and petrochemical industries, which are vital elements in many strategic value chains in the EU industry, are responsible for around 70% of industrial CO2 emissions (de Bruyn et al., 2020). 11% of industrial CO2 emissions in the UK come from steel production (Make UK, 2022).
In 2021, the European Commission presented the ‘Fit for 55’ package intending to reduce greenhouse gas (GHG) emissions by 55% by 2030, as compared with 1990, and to reach net-zero GHG emissions by 2050.
In March 2023, the European Commission proposed the Net-Zero Industry Act (NZIA), seeking to promote investments in the production capacity of products crucial to meet the EU’s climate neutrality goals.
The UK has set a target to lower carbon emissions by 68% by 2030, as compared to 1990 levels, and also has a target of 77% for 2035. In addition, the UK Government has committed to a 100% reduction of GHG emissions by 2050 compared with 1990 levels. Although both the EU and the UK have seen significant reductions in carbon emissions since 1990, it is clear that reaching net-zero emissions by 2050 – in less than 30 years – will require transformative changes to many societal activities, including industry.
Electrification is a key mitigation measure for industry
Electrification is a key measure for achieving deep emissions reductions in energy-intensive industries. This will typically require substantial process changes with high levels of investments and obviously entail an increase in electricity demand from both direct and indirect electrification systems (e.g., via hydrogen). In addition, the electricity sector has seen an increasing share of non-dispatchable electricity generation in the form of wind power (and some solar energy), which is expected to grow.
All electricity generation technologies face some challenges concerning social acceptance, and this is particularly the case for wind power in some regions, including Sweden, despite its vast land area, long coastline and low population density.
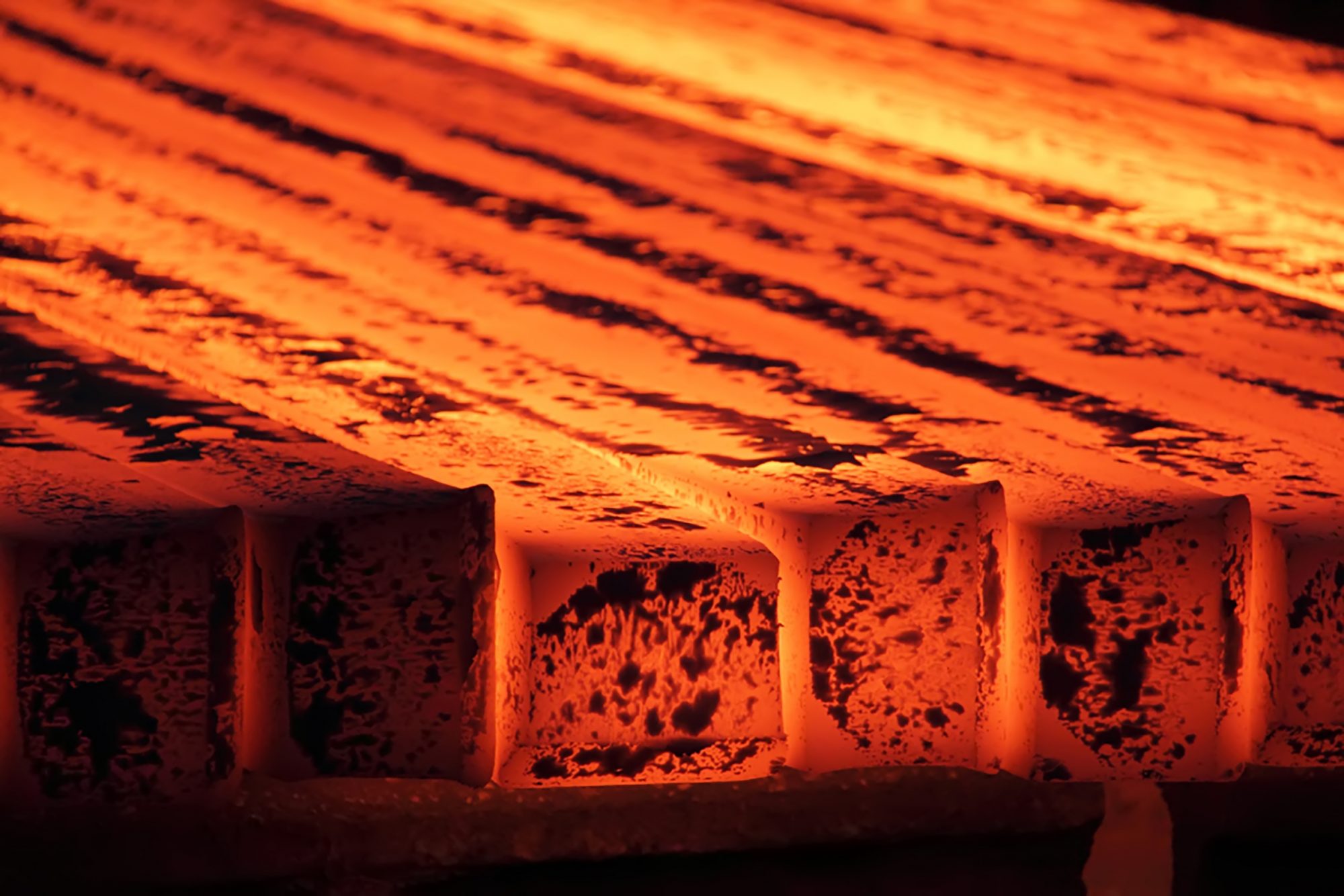
Three positive signs for the steel industry
However, at least three reasons exist to feel optimistic about current developments. First, there is increasing interest in decarbonising key value chains, including the steel industry. Many companies close to consumers, such as the automotive industries, have defined a target year for climate neutrality that includes the entire value chain (Scope 1 to 3 emissions). This means these industries must incorporate climate-neutral materials into their products, such as fossil-free steel in car manufacturing.
This is promising because it can contribute to mitigating the risk of high upfront investments in the basic material industry required for its decarbonisation. As an example, several industries (e.g., the automotive industry) in Sweden have signed letters of intent to purchase steel from the two fossil-free steel initiatives in Sweden: H2Green Steel and HYBRIT.
Second, there have been significant cost reductions in renewable electricity technologies, particularly for wind and solar power. This has resulted in the share of renewables in the EU’s final energy consumption increasing to 22% in 2021, compared with 9.6% in 2004 (Eurostat, 2023). This contributed to the carbon intensity of electricity generation declining from 400 gCO2/kWh in 2000 to 216 gCO2/kWh in 2020 (IEA, 2023).
The share of renewables in the EU is expected to increase, and the same trend is seen globally. The combined effect of rising carbon prices and decreasing prices for renewable energy and green hydrogen could make the electrification of steel production competitive within the next decade.
Third, there is a solid potential for implementing flexibility measures in the future electricity system, which could help to integrate large amounts of renewable, non-dispatchable electricity into the energy system (Göransson & Johnsson, 2018; Göransson, 2023). In other words, including flexibility measures will shift the electricity demand to maximise the utilisation of wind and solar power.
Such measures may include smart charging of electric vehicles, including Vehicle-to-Grid (V2G), smart control of heat pumps utilising the thermal inertia of buildings, and green hydrogen from electrolysers in combination with hydrogen storage for industry. In addition, enhancing the transmission grid at different voltage levels will even out the variability of electricity generation by reducing or eliminating congestion in transmission lines.
Flexibility in the electricity system is crucial for a successful transition
In our research, we investigate the possibilities associated with the electrification of industry. The research programs Mistra Electrification and Mistra Carbon Exit adopt inter-disciplinary approaches to investigate the drivers and barriers to decarbonising the steel industry’s supply chains, including the electrification of industry.
On the one hand, the three points mentioned above provide great possibilities for successful and efficient electrification, where, for example, hydrogen-based, fossil-free steel production combined with hydrogen storage would provide conditions for excellent wind power integration together with other flexibility measures in the form of smart charging of electric vehicles and control of heat pumps.
Transforming and decarbonising the steel industry in this way has the potential to change the European and UK steel industries from being importers of coal to instead using domestic energy and feedstocks (mainly electricity for hydrogen), while for some countries making it possible to export not only steel but also an intermediate product in the form of hot-briquetted iron.
As our research shows, this additional flexibility may also result in the re-location of future fossil-free steel plants, in that plant location will be determined not only by access to iron ore mining but also by the availability of low-cost electricity (e.g., Toktarova et al., 2021, 2022).
Challenges from non-technical barriers
On the other hand, the substantial need for investments in new electricity generation to power the electrification process faces challenges in the form of long permitting processes and low social acceptance. As indicated above, this is also the case in Sweden, despite its good conditions for low-cost electricity, with large land areas being available for new wind and solar power, combined with an already almost carbon-free electricity system based on hydro, nuclear and renewable electricity generation.
Need for solid political climate leadership & industrial policy
In addition, even though some customers have expressed willingness to pay a premium for fossil-free steel, governmental support for the transition of industry, including the steel industry, is most likely going to be necessary to mitigate the investment risk. This is because of the high upfront investment required at the same time as the urgency in scaling up fossil-free steel production if we are to follow a pathway for steel that is in line with the Paris Agreement (see examples of pathways in Toktarova et al., 2020).
Such support has been given in, for example, Germany and Sweden. Despite being much smaller than that in Germany and still pending, Swedish support has been questioned by some economists and investors. However, they do not propose any alternative way to meet climate targets.
There is a need for strong climate policy leadership to support the industries that want to meet climate targets. In the absence of such political leadership, there is a risk that those with vested interests in the fossil fuel industry will manage to delay the transition or that the transition will start elsewhere, leaving the EU and UK behind.
References
- de Bruyn, S., Jongsma, C., Kampman, B., Görlach, B., Thie, J-E. Energy-intensive industries – Challenges and opportunities in energy transition, Policy Department for Economic, Scientific and Quality of Life Policies Directorate-General for Internal Policies, July 2020.
- Make UK, 2022. UK Steel Net Zero Steel – A Vision for the Future of UK Steel Production. https://www.makeuk.org/about/uk-steel/net-zero-steel—-a-vision-for-the-future-of-uk-steel-production (accessed November 15, 2023).
- Eurostat. Renewable energy statistics 2022. https://ec.europa.eu/eurostat/statistics-explained/index.php?title=Renewable_energy_statistics#Share_of_renewable_energy_more_than_doubled_between_2004_and_2020 (accessed December 28, 2022).
- Göransson L. Balancing Electricity Supply and Demand in a Carbon-Neutral Northern Europe. Energies. 2023; 16(8):3548. https://doi.org/10.3390/en16083548 .
- Göransson, L., Johnsson, F. A comparison of variation management strategies for wind power integration in different electricity system contexts (2018) Wind Energy, 21 (10), pp. 837–854.
- IEA, 2023. Development of CO2 emission intensity of electricity generation in selected countries, 2000-2020 2022. https://www.iea.org/data-and-statistics/charts/development-of-co2-emission-intensity-of-electricity-generation-in-selected-countries-2000-2020 (accessed November, 2023).
- Toktarova, A., Walter, V., Göransson, L., Johnsson, F. Interaction between electrified steel production and the north European electricity system (2022) Applied Energy, 310, art. no. 118584. DOI: 10.1016/j.apenergy.2022.118584.
- Toktarova, A., Göransson, L., Johnsson, F. Design of clean steel production with hydrogen: Impact of electricity system composition (2021) Energies, 14 (24), art. no. 8349, DOI: 10.3390/en14248349
- Toktarova, A., Karlsson, I., Rootzén, J., Göransson, L., Odenberger, M., Johnsson, F. Pathways for Low-Carbon Transition of the Steel Industry—A Swedish Case Study (2020) Energies, 13 (15), art. no. 3840, DOI: 10.3390/en131538

This work is licensed under Creative Commons Attribution-NonCommercial-NoDerivatives 4.0 International.