The Center for Electron Nanoscopy at DTU discusses research into the magnetization of nanoparticle superstructures, advancing their use in modern technology
Magnetic materials at various length scales are everywhere in modern technology. From cars, trains, airplanes and wind turbines (1 mm – 1 m), to cellphones, computers, memory drives and loudspeakers (1 μm – 1 mm), down to spintronic devices and magnetic nanoparticles in biomedicine (1 nm – 1 μm).
Just as macroscopic properties of materials such as elasticity, conductivity, hardness, etc., emerge from specific arrangements of various atomic species glued by electric charges, magnetic properties such as coercivity, permeability and energy product are dictated by how elementary magnetic moments (spins) arrange and interact with each other at the atomic scale.
Nature provides us with a basic set of magnetic materials featuring different characteristics. Iron is “soft” (easy to magnetize), strong (producing a large field when magnetized), but prone to power losses. Magnetite, the natural iron-oxide compound where magnetism was first identified, is weaker but with lower power losses. Then we have Nickel, Cobalt, and rare-earths, each with a characteristic set of features that we can exploit in technological applications. Chemistry broadened the range of properties: synthetizing the NdFeB compound gave us a powerful magnet, which is so “hard” (difficult to magnetize, but also very persistent once magnetized) that can be used as “permanent” magnet in specific applications.
What if need a magnet that combines the strength of hard magnets with flexibility of manipulation and low power losses? Or a non-magnetic material that acquires magnetism upon compression? Answering this kind of questions required ingenuity, and that is how magnetic nanoparticle superstructures became the focus of scientific research over the past two decades. If we engineer new materials in a bottom-up approach where we substitute elementary spins with the macro-spin of magnetic nanoparticles, arrange them orderly at the nanoscale in chains, monolayers or 3D “super”-crystals, we can then fine-tune their interactions and drive the unique phenomenology that emerges [1].
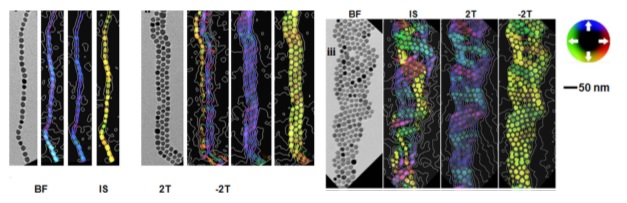
To fully understand the role of particle size, composition, and arrangement in determining the magnetic properties of superstructures [2], we need an imaging technique that can capture the distribution of the magnetic moments as we vary external parameters (applied field, temperature, chemical concentrations, etc.). Since nanoparticles are typically 10-15 nm in diameter, neither optical nor neutron-based techniques are adequate to achieve the single-particle resolution necessary to expose the spin topography. A technique called electron holography [3] is, instead, especially appropriate, as it combines high spatial resolution (1-5 nm) and sensitivity to tiny magnetic fluxes (down to 1/200 of a flux quantum).
We have used electron holography to study the interplay between dimensionality of the superstructure (1D chains to quasi-2D granular strips), type of “super”-lattice (e.g. square vs. close-packed), geometrical disorder, and magnetic order [4]. Figure 1 shows transmission electron microscopy (TEM) bright-field images of 15-nm ε-Co particle assemblies, and the corresponding magnetic moment distribution retrieved with electron holography. We observed that chains wider than 1 particle across are preferentially assembled into close-packed lattices, although square lattice arrangements are also occasionally seen. The particles are separated by at least 2 nm (the size of the surfactant layer) providing full exchange decoupling and leaving dipolar interactions as dominant.
Our analysis revealed that despite the absence of exchange interactions, which are normally the main driving force behind ferromagnetism, the magnetization of nanoparticle superstructures is very resilient: we observed long-range magnetic correlations even when the structures were so disordered to make them essentially amorphous. These observations contrast with the magnetic glass behaviour found in random 3D particle arrangements [5] and are of direct relevance for the use of quasi-2D nanoparticle assemblies in magnetic devices such as thin elongated memories, tunnel junctions, sensors, and other magnetic devices where properties may be engineered and fine-tuned from the bottom up.
References
[1] M. Varon et al., Scientific Reports 5, 14536 (2015)
[2] M. Kure, M. Beleggia, C. Frandsen, J. Appl. Phys. 122, 133902 (2017)
[3] A. Tonomura, Rev. Mod. Phys. 59, 639 (1987)
[4] M. Varon et al., Scientific Reports 3, 1234 (2013)
[5] S. Mørup, G. Christiansen, J. Appl. Phys. 73, 6955 (1993)
Associate Professor Marco Beleggia
Center for Electron Nanoscopy
DTU Cen, Technical University of Denmark
Tel: +45 4525 6494
mb@cen.dtu.dk