Discovering cures for human diseases or how to recover from damage or trauma to tissues is the fundamental goal of medical researchers, but it is a daunting task. Nevertheless, recent studies have shown the remarkable potential of the spiny mouse in addressing these crucial issues
How can we induce the regrowth of myelin to stop the debilitating effects of multiple sclerosis? How can we induce the regeneration of cardiomyocytes after a heart attack or the regrowth of axons after spinal cord damage?
The classical approach to this regrowth problem in mammals is to study a model organism that can recover from this type of degeneration or trauma and learn how they do it.
Following the hypothesis that regenerative mechanisms are conserved throughout the vertebrates, we could then extrapolate these finding to develop therapies for humans. Traditionally, lower vertebrates such as zebrafish, newts and salamanders have provided model systems with which to study tissue regeneration and many important insights have come from these studies. However, we have recently discovered an adult mammal that can already regenerate several of its tissues and organs, so studying this organism will allow us to speed up the therapeutic discovery process.
This organism is the spiny mouse of the genus Acomys. We have shown that it can perfectly regenerate a hole punched through the ear and after removing large pieces of skin. In these instances, the epidermis, dermis, hairs, sebaceous glands, erector pili muscles, cartilage and skeletal muscle of the panniculus carnosus regenerate.
The skin can also regenerate perfectly after a burn injury, the kidney and spinal cord have greatly reduced fibrosis after damage, which permits improved recovery, and so does the heart after a myocardial infarction.
The spiny mouse can thus regenerate each of the three types of muscle: smooth, skeletal and cardiac and here I describe the circumstances under which this may provide some important avenues for extrapolation to humans.
Smooth muscle: In the dermis, there are two structures that contain smooth muscle cells, namely the vasculature and the muscles that raise hairs when we are cold or frightened.
Cutting out a piece of skin removes both of these smooth muscle tissues (erector pili muscles Fig 1A stained with smooth muscle actin) and in the spiny mouse, the newly regenerated skin contains both of these structures, which have regenerated anew (regenerated erector pili muscle Fig 1B).
The lab mouse (or human) only scars and no hairs are regenerated so there will obviously be no regeneration of these erector pili muscles. This suggests that in the spiny mouse, the regeneration of smooth muscle erector pili muscles is induced by the new hair follicles and unravelling the molecular mechanisms (for example, which growth factor induces smooth muscle differentiation) and cellular origin (dermal fibroblasts or hair follicle stem cells) will be an important avenue for further discovery. This may lead to ideas for treatments for diseases in which there is smooth muscle degeneration in the lung, the gut or the bladder, for example.
Skeletal muscle: When the full thickness skin is removed, the skeletal muscle layer at the bottom of the skin, known as the panniculus carnosus, is also removed. This type of injury, where a hole is created in skeletal muscle, normally creates permanent damage in mammals because skeletal muscle needs a connective tissue sheath to induce or guide its regeneration. This is known as a volumetric muscle loss.
This defect is not regenerated in the lab mouse but in the spiny mouse, amazingly, the defect is regenerated and embryonic myosins and other myogenic transcription factors are induced again in a recapitulation of development (Fig 1C). Being able to induce the regeneration of a volumetric muscle loss in humans after trauma would be of major significance.
Lab mouse skeletal muscle can regenerate, however, if the connective tissue surrounding the muscle fibres is not removed and the muscle is injected with snake venom. Under these conditions, the skeletal muscle stem cells, the satellite cells, proliferate and redifferentiate back into myonuclei.
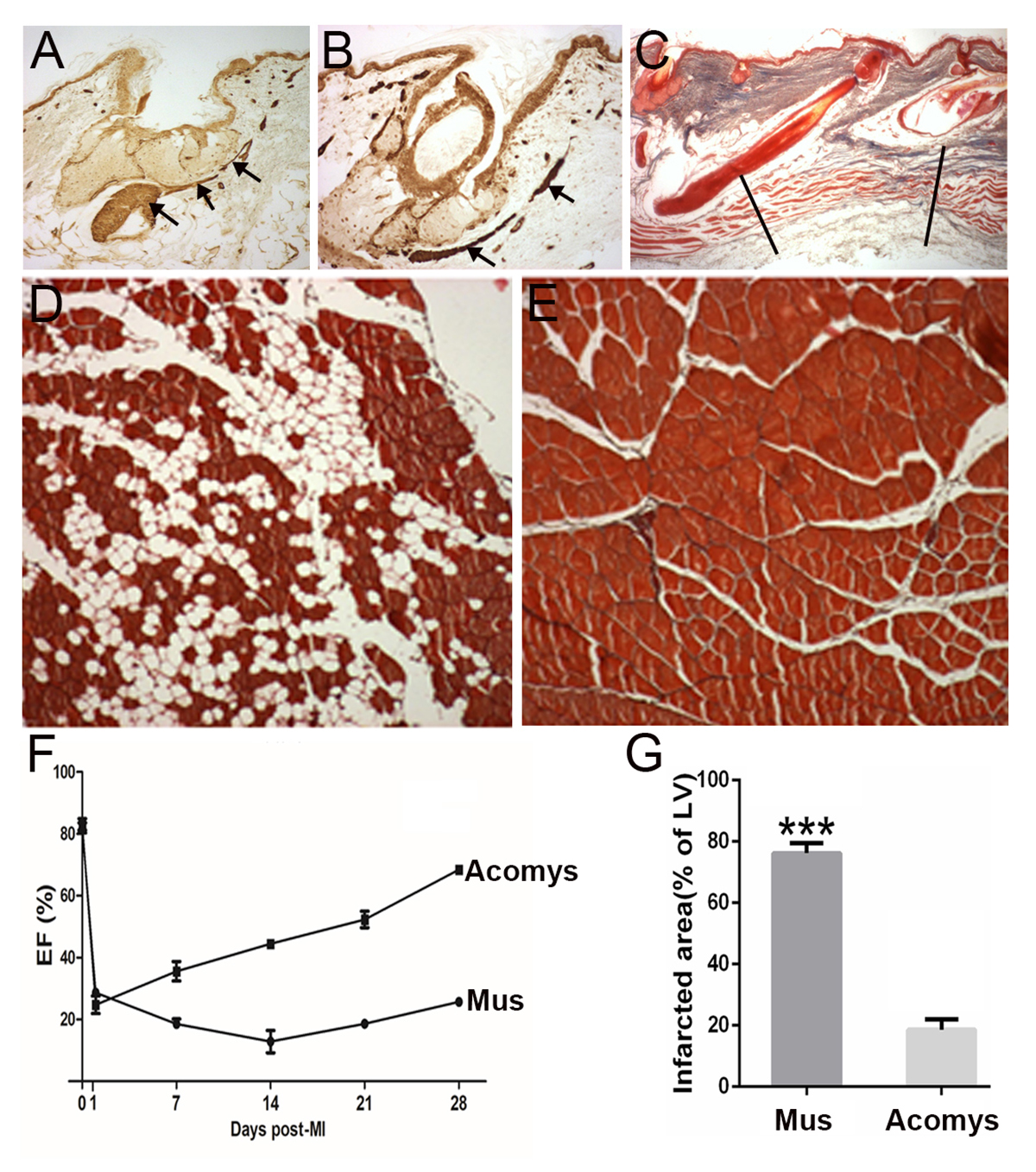
When we repeat this regenerative process five times then the lab mouse muscle starts to fail in its regenerative capacity, probably because it runs out of stem cells and gradually replaces the muscle fibres with fat tissue (Fig 1D).
In contrast, the spiny mouse muscle after repeated rounds of regeneration will continue to perfectly replace myofibres without any signs of fat tissue (Fig 1E).
In the disease of muscular dystrophy, the affected muscles are in a permanent state of regeneration and the myofibres gradually fail to be replaced by more muscle and fat regenerates instead exactly in the case of the repeated regenerating lab mouse (Fig 1D). As a result of the replacement of myofibres with fat, the performance of the muscle in humans declines over time and produces the terrible muscular wasting we see with this disease. Learning how to perfectly regenerate muscle fibres repeatedly as the spiny mouse can (Fig 1E) may have important implications for the treatment of muscular dystrophy.
Cardiac muscle: Following a myocardial infarction (heart attack), the lab mouse and the human heart undergoes a wave of cardiomyocyte cell death and the fibroblasts at the damaged site lay down a collagenous scar. The result is a reduced pumping power, measured as ventricular ejection fraction (Fig 1F).
In an attempt to counteract this loss, the remaining ventricle wall thins and expands, making itself much more liable to a further damage.
Remarkably, the spiny mouse heart after a myocardial infarction rapidly recovers its pumping performance in terms of the ejection fraction (Fig 1F) and there is a vastly reduced scar present at the site of damage (Fig 1G). It effectively regenerates its cardiomyocytes after a heart attack and discovering the molecular basis of this would have a huge impact on human health as this is the biggest killer in the Western world.
We can see that the adult spiny mouse is a remarkable animal model that can regenerate each of its three types of muscle, as well as more complex structures. Discovering the reason for this property may provide some answers to very significant human healthcare problems.
Please note: this is a commercial profile
Malcolm Maden
Professor
Department of Biology & UF Genetics Institute
Tel: +1 352 273 7875