Takayuki Nonoyama from Hokkaido University in Japan focuses on an aspect of materials science behind the soft matter that possesses a rubbery-to-glassy transition in a novel polymeric hydrogel material, contrary to the intrinsic property of polymers
From the experience of plastic bottles and bags softening at high temperatures, conventional polymers are turned into a hard/glassy state and soft/rubbery state at low and high temperatures, respectively.
The critical temperature is called the glass transition temperature (Tg). Around the Tg, the elastic modulus drastically changes by around three orders of magnitude that are corresponding to the change from food jelly to resin. Since the change is universally observed regardless of the type of polymer, the glassy-to-rubbery transition is an intrinsic property of polymers. While this property contributes to enabling the high-speed mass production of polymer products, the softening at high temperature causes deterioration of fuel efficiency with a decrement of tyre performance. Until 2020, marking the 100th anniversary of the start of polymer science advocated by Hermann Staudinger in 1920, there have been no reports of polymers that vitrify and harden at high temperatures due to state changes, not because of irreversible reactions such as thermosetting resins.
A novel polymeric hydrogel material
I incidentally discovered a polymeric hydrogel material that possesses a novel reverse glass transition that is “rubbery-to-glassy transition with elevating temperature”, while undertaking a completely different area of research (Figure 1).
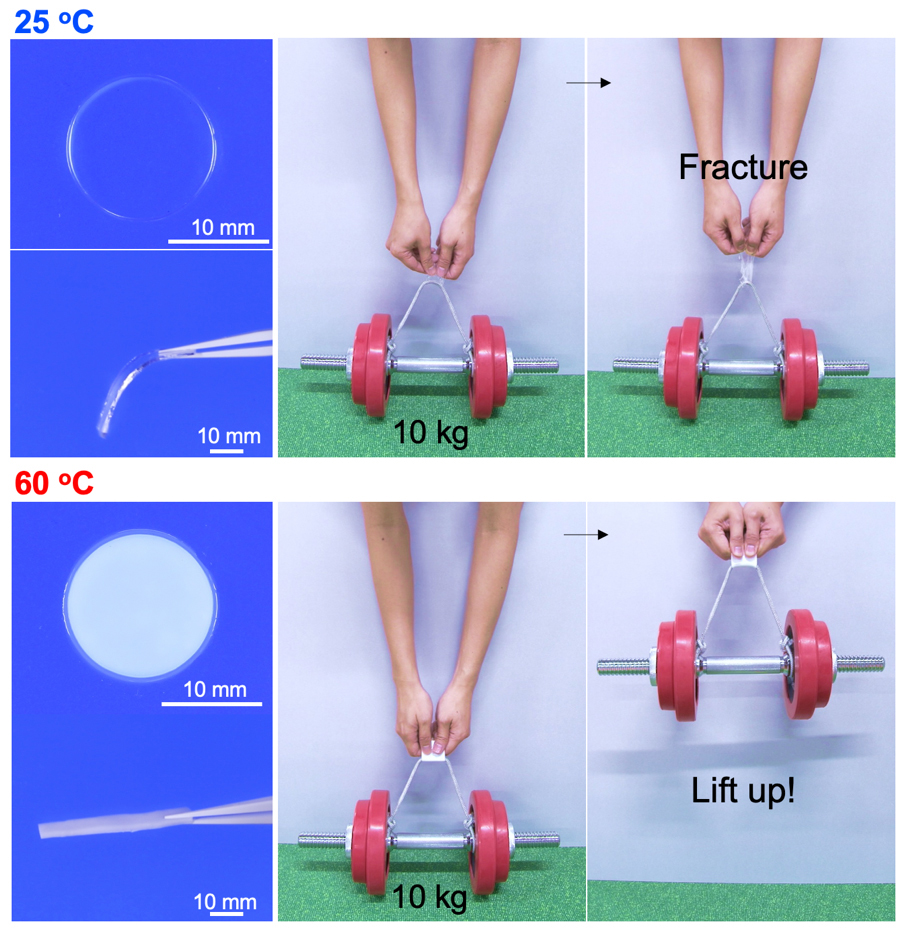
The soft and transparent gel sheet cannot lift up the weight at room temperature, however, while it becomes stiff and turbid, it can lift up. This material, consisting of poly (acrylic acid) hydrogel equilibrated in calcium acetate solution, achieves the reverse glass transition by utilising the thermal phase separation. Both are versatile, inexpensive, and non-toxic chemicals that are used as a food additive. In conventional phase separation of a hydrogel, the gel has a homogeneous one-phase at low temperatures in which polymer network is hydrated well.
When the temperature elevates beyond the phase separation temperature, the polymer is dehydrated and separates into the polymer dense phase and dilute phase (water phase). Many such temperature-responsive phase-separated gels have been reported, but the most variable factor is that our gel exhibits “extremely strong phase separation compared to other phase separation gels”. Since the phase separation of the conventional gels is relatively weak and the density of the polymer in a polymer dense region does not increase so much, water molecules that act as a plasticiser for the hydrophilic polymer are present in the dense phase. Even after the phase separation, the dense region remains in a soft rubbery state.
On the other hand, our gel reaches an extreme phase separation state in which the polymer and water are almost completely separated. Since there is almost no water in the polymer dense state, the intermolecular interaction and entanglement between the polymers are extremely high, and the polymer finally reaches its glassy state. In addition, during the extreme phase separation, water molecules are not discharged to the outside of the gel matrix and form water clusters. In other words, in the macroscopic perspective, water is not lost, and the overall water content of the gel material didn’t change, while in the microscale, the dense phase forms a structure that is close to a completely dry state.
Novel features of the gel
Due to the unique phase separation, this gel exhibits the following novel features that are different from the conventional phase separation gels.
It shows a rubbery-to-glassy transition at an elevated temperature, which is the exact opposite phenomenon of conventional polymers, and a modulus transition of three digits or more is observed.
Since water is stored in the gel matrix, the volume does not change before and after phase separation.
The two also means that the moving distance of water molecules accompanying the phase separation is completed on a microscale, that is, the thermal response is extremely fast (vitrification in a few seconds).
Since it is caused by the phase transition, it recovers to the original homogeneous rubbery state when the temperature is returned, and the phase separation phenomenon can repeat many times.
A large heat absorption is accompanied. Apart from this material, there have been no other reports of polymers that instantly harden by three orders of magnitude or more without changing volume, and these materials have a large potential in applications.
Potential applications
Here, two potential applications are demonstrated. One is a thermo-active smart protector that assumes a traffic accident has taken place, which could involve motorcycles (Figure 2).
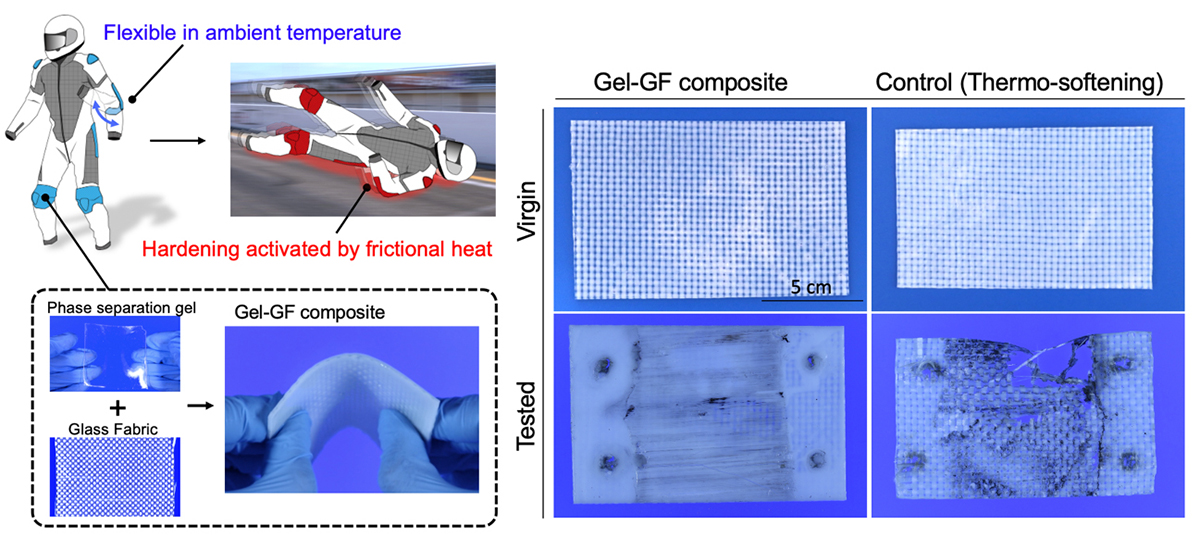
The phase separation gel combined with woven glass fibre fabric is soft and bendable not to constrict motion in normal temperature, but when it was pulled against an asphalt surface for five seconds at a speed of 80 km/h, it hardened by friction heat, with only minor abrasions forming on the contact surface. The surface temperature just after the frictional test reached 90ºC, which is much higher than the rubbery-to-glassy transition temperature of the gel (50ºC).
On the other hand, the thermo-softening gel – glass fibre fabric composite as a control sample is obviously fractured in the test. The other application is heat-absorbing sheets. An energy-free air-conditioning system has been required as a solution to prevent global warming. When the gel sheet with phase separation temperature of around 30ºC is attached to a glass window, the part of the radiant heat of sunlight is absorbed by the gel sheet due to phase separation and the rise of interior temperature can be reduced. Since the phase separation is reversive by changing temperature, this system can be repeated many times by a day/night cycle.
A unique achievement in conventional polymers
The greatest academic interest of this research is the phenomenon of “glass transition opposite to temperature”, which is unprecedented in conventional polymers. The intrinsic feature in which a polymer softens at a high temperature leads to, for example, increase deformation resistance of tyres and energy dissipation to heat, and is directly linked to problems such as deterioration of performance and fuel consumption. Although the rubbery-to-glassy transition at elevated temperature of this material, which was incidentally discovered, goes against the “soft” properties required for polymer materials, it has a great impact on society and the economy in that it can solve these problems in the future.
Please note: This is a commercial profile