The Henry Royce Institute is enhancing the UK’s position as an international leader in the field of biomedical materials, systems and devices
The Henry Royce Institute for advanced materials (Royce) is the UK’s national institute for advanced materials research and innovation. Operating across nine Partner Institutions, including the Royce Hub at The University of Manchester, we provide world-class equipment, infrastructure, training and outreach.
Royce is able to support healthy ageing by accelerating the translation of materials-led technologies, promoting collaboration, supporting research-foresighting, enabling skills development and providing flexible access to state-of-the-art research facilities. The breadth and quality of manufactured, characterised and tested materials is targeted to support progression through Technology Readiness Levels (TRLs) to de-risk technologies for industry and generate improved biomedical material products.
Globally the number of individuals aged over 60 is projected to reach 1.4 billion by 2030, leading to accelerating demand for curative and affordable materials-driven solutions. Tissue repair and regeneration is a complex physiological process that requires multiple biological and physico-chemical cues acting together.
Biomedical materials product innovation
As one of the leading Royce Partners, The University of Manchester has held workshops and conferences to engage the research community as a whole to work towards current clinical need. Biomedical materials solutions and innovations hold promise to improve healing outcomes in a cost-effective and scalable manner. Two case examples of the development and translation of bio-interactive materials are highlighted.
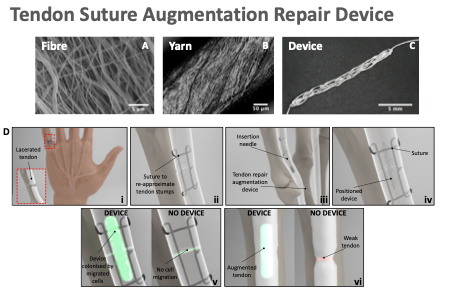
Case study 1: Degradable materials for novel tendon repair strategies
Professor Sarah Cartmell is leading a team in developing a portfolio of new degradable tendon repair devices that aim to reduce the repair time of injured tendons and improve functional repair outcome. She has been collaborating with clinicians such as Mr Jason Wong (Manchester University NHS Foundation Trust) to ensure that this technology is clinically relevant.
One of these products is a tendon repair device that can be used to augment the current suturing technique for injured tendons; the suture provides the physical connection between the ends of the tendon; the degradable nano-scale fibrous product acts as a ‘bridge’ for cells to migrate over to improve functional tendon repair (Figure 1). This technology is currently being translated by NLC – the European healthtech venture builder https://nlc.health.
Case study 2: Electrically conductive materials for improved nerve repair
Electrically conductive materials are emerging as important biomedical materials in medical products. Bioelectronics is a rapidly developing area of research at the interface between electronics and living systems for creation of sensory, stimulatory or responsive devices.
Physiologically, the human body is controlled by electrical signals in the central nervous system and the peripheral nervous system (PNS). Disruption of PNS by trauma or disease can lead to loss of sensation and movement and significant socio-economic cost. Work at The University of Manchester has focused on developing novel conductive materials that have nano-scale fibrous structures to stimulate and guide excitable neuronal cells and nerve tissue repair. The materials are based on conductive polymers, such as poly (3,4-ethylenedioxythiophene) polystyrene sulfonate (PEDOT:PSS) coatings on electrospun silk fibroin fibres. The fibrous ‘scaffold’ provides physical guidance to nerve cells as they grow (proliferate and elongate along fibres) and connect, as well as offering the potential to conduct electrical signals between cells leading to effective peripheral nerve repair (Figure 2).
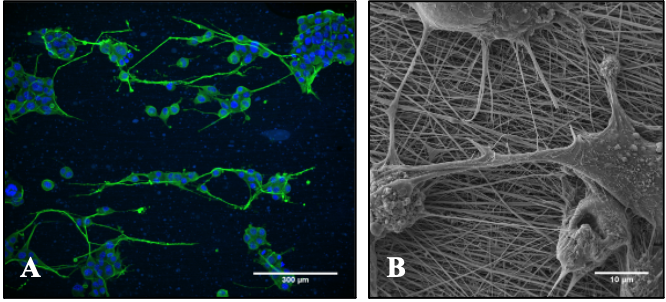
Future vision
The future of research at Royce will include innovation of further products and medical solutions through engagement with our academic, clinical and industrial collaborators. Working with these stakeholders to expand the use of our facilities and associated access schemes to accelerate research outputs, as well as to broaden the opportunities for access.
We are training our next generation of researchers to ensure capability improvements and to build the skills base. One way in which this is occurring is through recently funded Engineering and Physical Sciences Research Council (EPSRC) Centre for Doctoral Training (CDT) in Advanced Biomedical Materials. The CDT is held by Universities of Manchester and Sheffield and currently training over 50 new doctoral students.
To conclude, biomedical materials is an exciting, fast-moving and much-needed research area. With our current interdisciplinary approaches at Royce, as well as our knowledge of the regulatory and clinical/industry roadmap needed to deliver product development and deployment, we have the potential to make a huge impact on the patient quality of life and can help address the needs of our increasing and ageing population.
Working with the materials community, Royce identifies the following research areas:
- Translation of bio-interactive materials expanding the applications and innovation of existing approved materials and development of new bio-interactive materials.
- Affordable materials solutions for sustainable health improving implant efficacy and lifespan, digital health monitoring and rapid diagnostics for early and effective treatments.
- Development of tissue analogues in vitro synthetic cell-microenvironments to study interactions and develop novel regenerative therapies.
- Materials for a safer world anti-viral/anti-microbial materials and surfaces.
Acknowledgement: This work was supported by the Henry Royce Institute for Advanced Materials, funded through EPSRC grants EP/R00661X/1, EP/S019367/1, EP/P025021/1 and EP/P025498/1.
Please note: This is a commercial profile
© 2019. This work is licensed under CC-BY-NC-ND.