The Fundamental Pieces of Visible Matter: Offering an Unprecedented Insight into the 3D Structure of Hadrons and the Proton Spin Puzzle
All the visible matter in the universe is composed of atoms, which consist of electrons orbiting a small but extremely dense nucleus. The nucleus contains protons and neutrons, which also have a substructure of particles called quarks. Quarks come in different types, called flavors: up, down, strange, charm, bottom, and top. The quarks are held together by the strong nuclear force, which is mediated by other particles called gluons. Quarks and gluons, collectively called partons, are some of the most elementary pieces of visible matter in the universe. A “zoo” of particles, generically called hadrons, is made up of quarks and gluons.
“Seeing” the Structure of Hadrons through “High-Energy Microscopes”
Since quarks and gluons exist at extremely small distances inside of hadrons, physicists must use very high-energy collisions to probe their structure. Electrons and protons can be accelerated close to the speed of light and smashed into other protons in order to break them apart and “see” the partons inside. However, due to a feature of the strong nuclear force called confinement, partons are never found in isolation but always bound inside of hadrons. Analyzing the aftermath of these collisions allows for physicists to reconstruct the initial state and study the inside of the proton.
This requires an interplay between experimental measurements and sophisticated theoretical frameworks from which information about hadrons can be extracted. Over the course of the next decade, a new collider, called the Electron-Ion Collider (EIC), will be constructed at Brookhaven National Lab in the United States. The EIC is a project involving thousands of physicists worldwide that will offer unprecedented insight into the structure of hadrons (1).
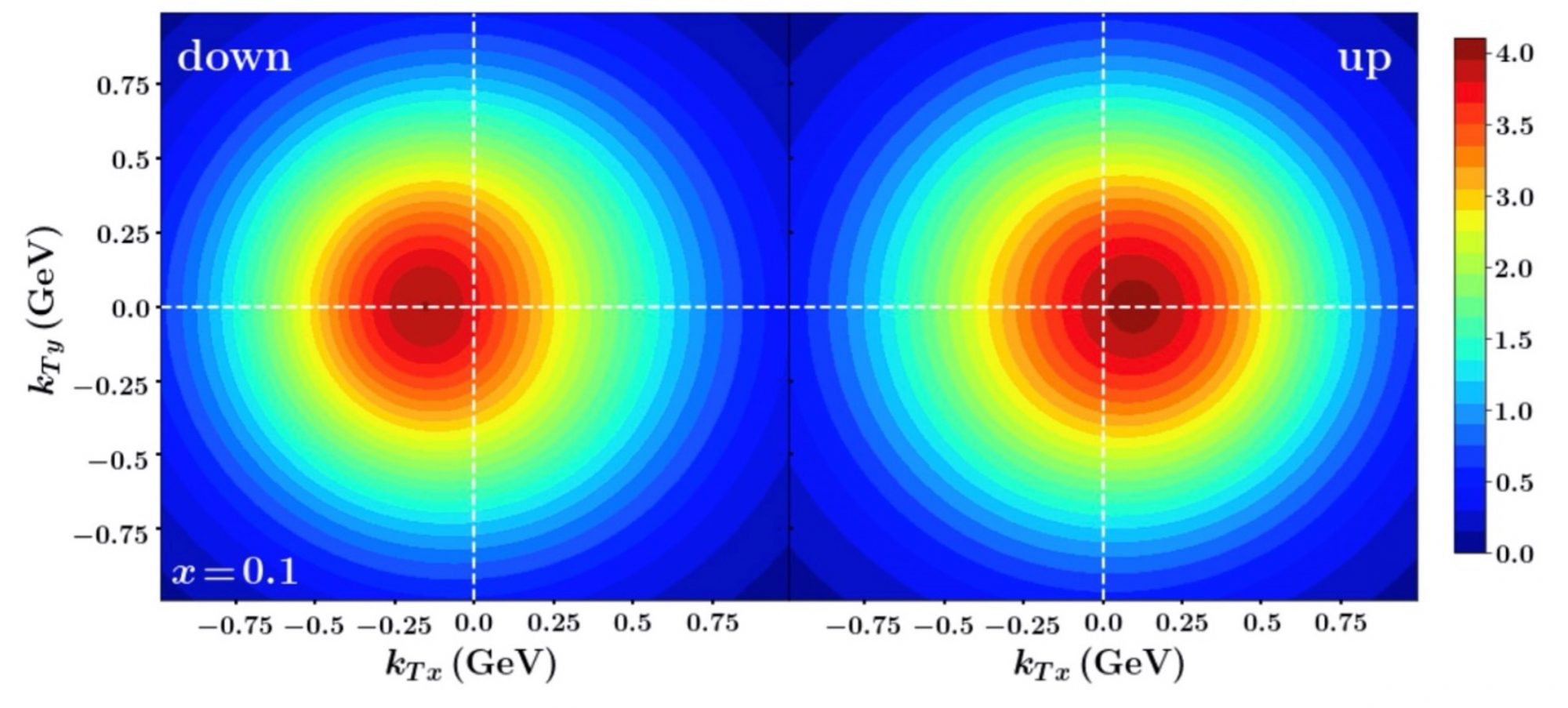
The x- and y-components of kT are shown along with the likelihood for a quark to carry that momentum (indicated by the different colors, with the scale given by the bar on the right).
The proton has its spin oriented in the y (vertical) direction and is moving towards the observer.
Hadron Tomography
Hadrons are a complex system of quarks and gluons moving around and interacting with each other. If we consider the case of a proton moving with a momentum P, then each parton inside carries a certain fraction x of this momentum. However, partons do not just move in the same direction as the proton, but they also have an intrinsic momentum kT transverse (perpendicular) to the proton’s momentum. This 3-dimensional (3D) structure is encoded in so-called transverse momentum dependent parton distribution functions (TMD PDFs) that depend on (x, kT). These functions allow us to take tomographic slices of hadrons to see how quarks and gluons move around inside.
Critical to understanding the 3D (momentum) structure of hadrons is studying experimental observables sensitive to the spin of the proton. Spin is an intrinsic angular momentum (rotation) that a particle has (although it is not literally rotating). If the proton is unpolarized (spin is averaged over), the quarks are symmetrically distributed inside. However, if the proton’s spin is oriented transverse to its momentum, we find a distortion in where the quarks are located. By extracting a TMD PDF called the Sivers function (2), we can create an image (in momentum space) for a given value of x of the likelihood for a quark to move with a certain value of kT – see Figure 1. The results show that, if the proton moves towards the observer, the up quarks shift off to the right and the down quarks to the left. Future experimental data from the EIC will allow for more precise tomographic images of the proton’s internal structure.
The Proton Spin Puzzle
As previously mentioned, particles have an intrinsic angular momentum called spin, which is a fundamental property of the particle. The proton is a spin-1/2 particle, meaning its spin equals h/4π, where h is Planck’s constant. Since the proton is composed of quarks and gluons, its spin should be a sum of these partons’ spins and orbital angular momenta (OAM). Quite surprisingly, only about 30% of the proton’s spin comes from the quark spins. The gluon spins contribute about 30-50%, and the rest is thought to be from the OAM of the quarks and gluons. However, more experimental measurements and theoretical analyses are needed to precisely pin down how the pieces of the proton spin “puzzle” fit together. This is one of the main goals of the future EIC.
This work was supported by the National Science Foundation under Grant No. PHY-2011763.
References
- R. Abdul Khalek, et al. “Science Requirements and Detector Concepts for the Electron-Ion Collider: EIC Yellow Report,” Nucl. Phys. A 1026, 122447 (2022).
- J. Cammarota, L. Gamberg, Z. B. Kang, J. A. Miller, D. Pitonyak, A. Prokudin, T. C. Rogers and N. Sato, “Origin of single transverse-spin asymmetries in high-energy collisions,” Phys. Rev. D 102, 054002 (2020).

This work is licensed under Creative Commons Attribution-NonCommercial-NoDerivatives 4.0 International.