Thomas J Webster, Ph.D., Professor and Entrepreneur, is investigating the potential of AI in medical applications and biomaterial production
What is Artificial Intelligence?
According to the Webster’s dictionary definition, Artificial Intelligence (AI) is: a branch of computer science dealing with the simulation of intelligent behaviour in computers, and/or the capability of a machine to imitate intelligent human behaviour. (1)
Much has already been said about AI and, unfortunately, most of it is negative. (2) From students using ChatGPT to write term papers and college admission essays to AI self-driving cars that can crash and cause injuries (3), AI has certainly received a lot of negative press lately. As usual, however, some of this negative press is by those who are not scientists and do not understand the positive impacts AI can have, especially in medicine.
During COVID-19, we have all become accustomed to false reporting about groundbreaking science and scientists and need to be cautious of such information. We should, instead, learn for ourselves. Professor Thomas J Webster is one of those learning for himself the value of AI in improving medicine, if used appropriately.
He has been exploring how AI can improve medicine, and, in particular, improve the design, fabrication, and use of biomaterials across all of healthcare. To ensure such benefits reach the patient, Professor Webster has been commercialising AI in medicine by starting new companies in a new field he has coined ‘BandAId™’ (Figure 1).
The conventional way biomaterials have been developed
It is clear that very little innovation in biomaterials has occurred over the past century. In fact, as just one example, we are still implanting today the same hip implant (in terms of materials and geometry) originally designed by John Charnley in 1962. (4) Yet, we are asking more from these implants: inserting them into a wider range of patients, and expecting them to perform longer and more robustly. Furthermore, they are being challenged by an ever-growing population of antibiotic-resistant bacteria. This has got to stop.
Conventionally, whether speaking of orthopaedic devices or those used for cardiovascular applications, research for new implants has included trial-and-error in vitro and in vivo testing for better material chemistries. These are chemistries that can reduce infection, reduce platelet activation, promote appropriate tissue growth, and limit chronic inflammation.
Sometimes, research has centred on selecting the best mechanical properties for that specific chemistry for a particular medical device application. While countless start-up companies have formed from such approaches, they have not in general led to the well-oiled machine improving life expectancy. (5) Note that life expectancy was decreasing in parts of the world even before COVID-19, highlighting the failures of this conventional approach to new medical device design. (6)
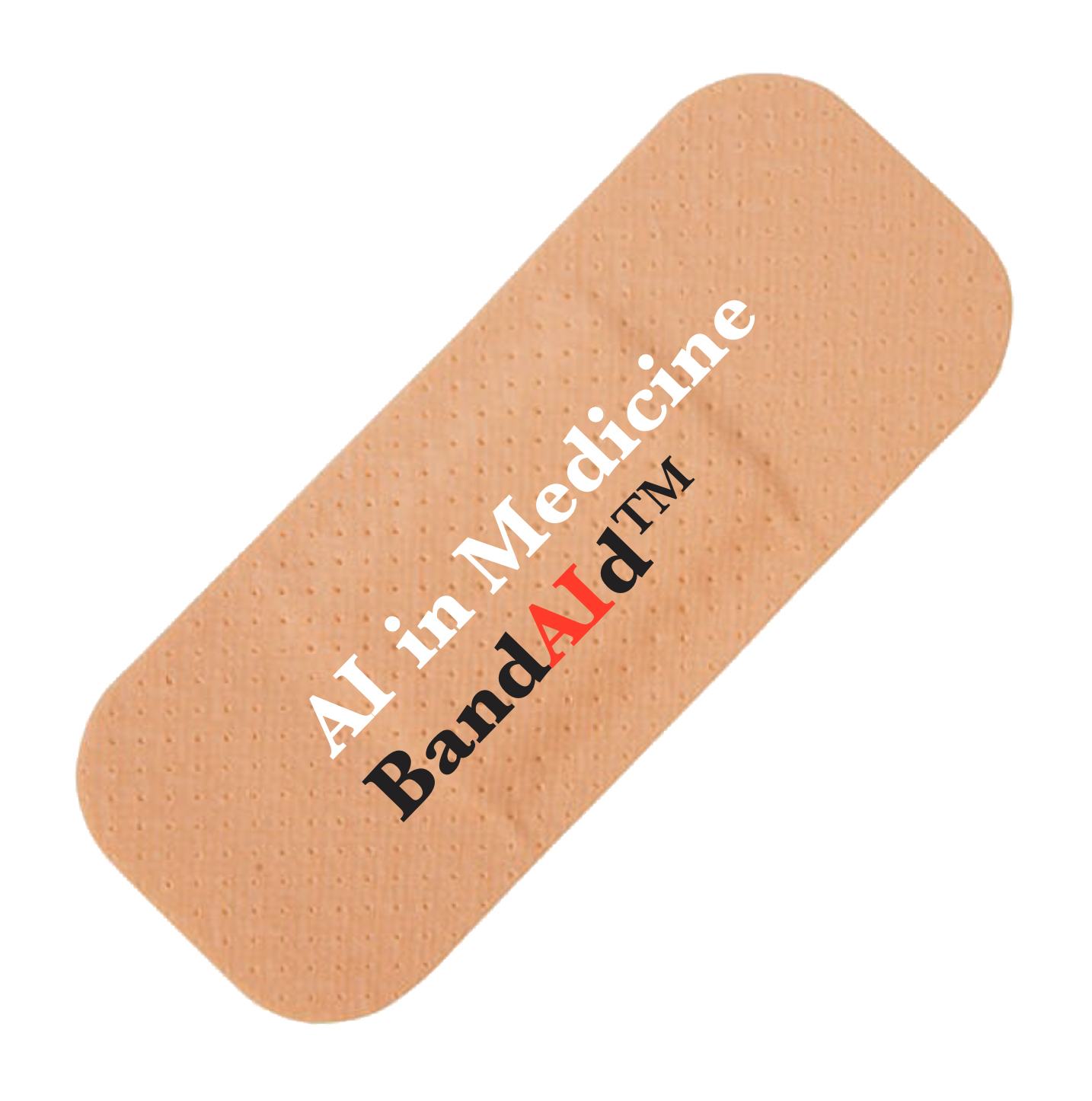
The BandAId™?
What has been forgotten in traditional biomaterial design is that patients will respond differently to the same implant. Why is it that the same implant inserted the same way and using the same procedures will sometimes lead to implant failure and will sometimes lead to implant success? What is different? The difference is the patient and their immune system. We know that a person’s immune system gets weaker as they age.
We know that females and males have different immune responses. We know that active patients rebound from injury quicker than less active patients due to altered immune systems. And we know that two perfectly healthy individuals have different immune system responses to the same material.
So, when inserting a foreign body into a patient to rebuild bone, open up clogged arteries, straighten a spine, and more, why don’t we consider the various immune systems that change from patient to patient? This is where Professor Webster’s vision for AI comes in.
Professor Webster and his team have developed numerous biomaterials that use AI to understand patient history and, based on that information, predict how that patient will respond to an implant. No more trial-and-error biomaterial design. And, most importantly, unlike traditional academic research, he is translating such research into real commercial products destined to eliminate medical device failure indefinitely. Let’s give some examples.
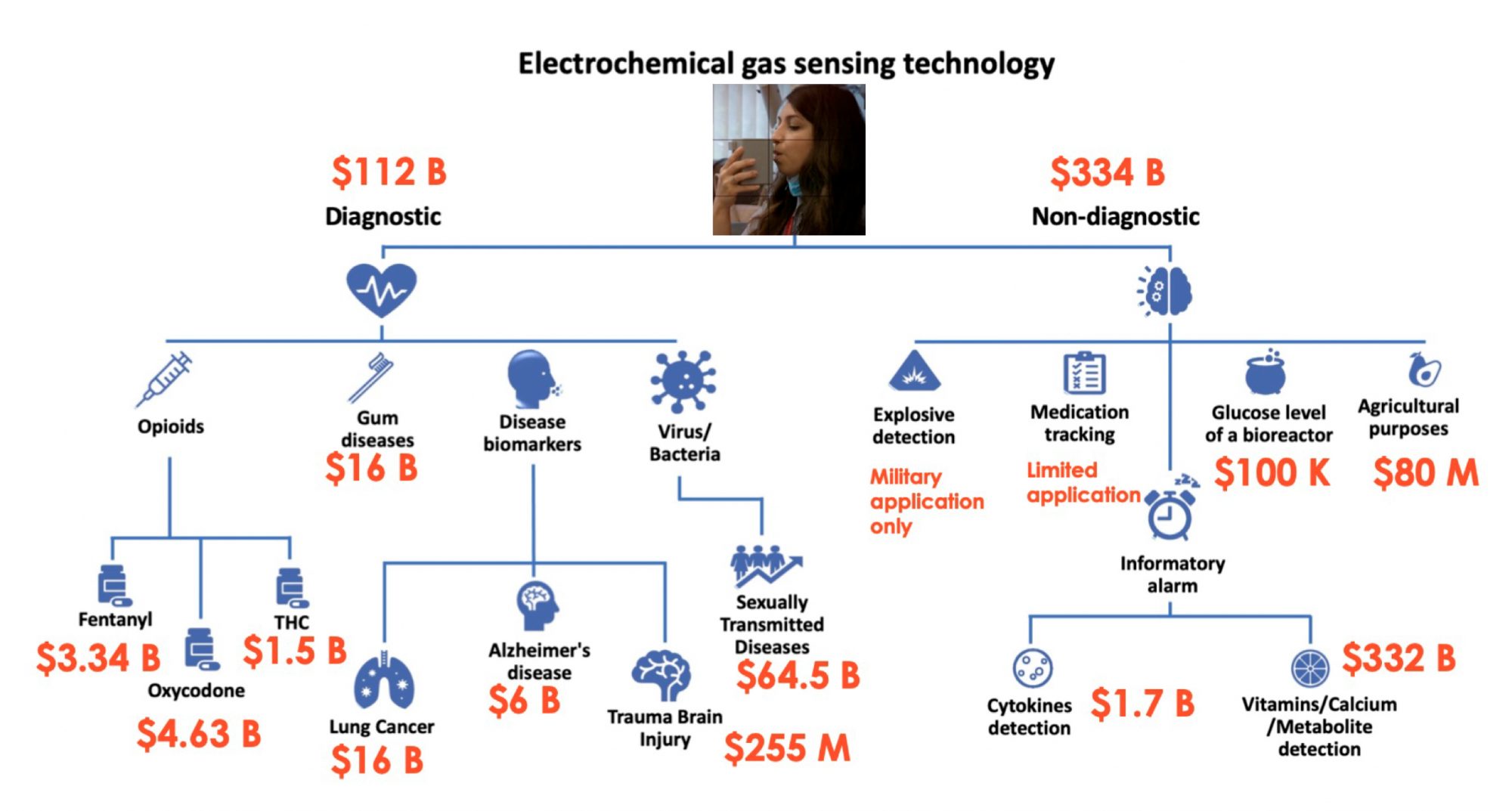
BandAId™ Examples
A company Professor Webster is involved in has developed a breathalyser that can detect analytes in one’s breath to diagnose everything from Alzheimer’s disease, infections, sexually transmitted diseases, COVID-19, and more (Figure 2). Quarksen is revolutionising sensor use and has thus made it possible to easily track disease treatment through individuals’ breath. They are incorporating AI algorithms to interpret data collected from such breathalysers and predict better treatments.
As another example, Professor Webster and his team have developed the concept of ‘Nano-optimizedTM’ where, based on prior implant success or failure and accompanied immune response, one can implement nanotextured features through AI to either ‘turn-on’ or ‘turn-off’ the immune system (Figure 3).
Or one can develop nanotextured features to inhibit bacteria colonisation if the patient has a weakened immune system or a history of implant infection. An example in Figure 3 is provided where tendon fixation devices were Nano-optimized to promote tendon growth, whereas today’s tendon fixation devices simply mechanically fix such tendons into bone. This is perfect technology for a patient with a history of orthopaedic soft tissue injuries.
Figure 4 highlights Kalnar Technologies in which Professor Webster has used his expertise to grow sensors from implants and, by gathering information of what types of cells are attaching to implants in real-time, use AI to predict whether implant infection, chronic inflammation, or appropriate tissue growth will occur. Demonstrated in this figure is how such sensors can be created by atomic layer deposition (ALD) to possess a nanoscale topography to promote bone growth in the spine of sheep after 12 weeks.
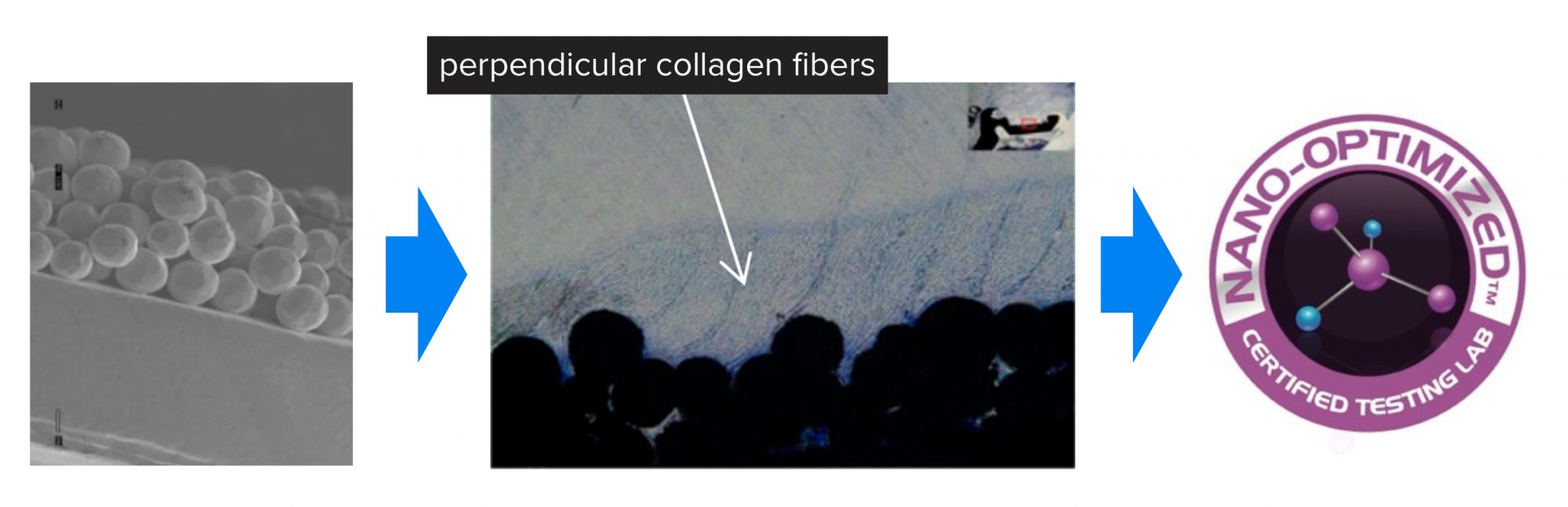
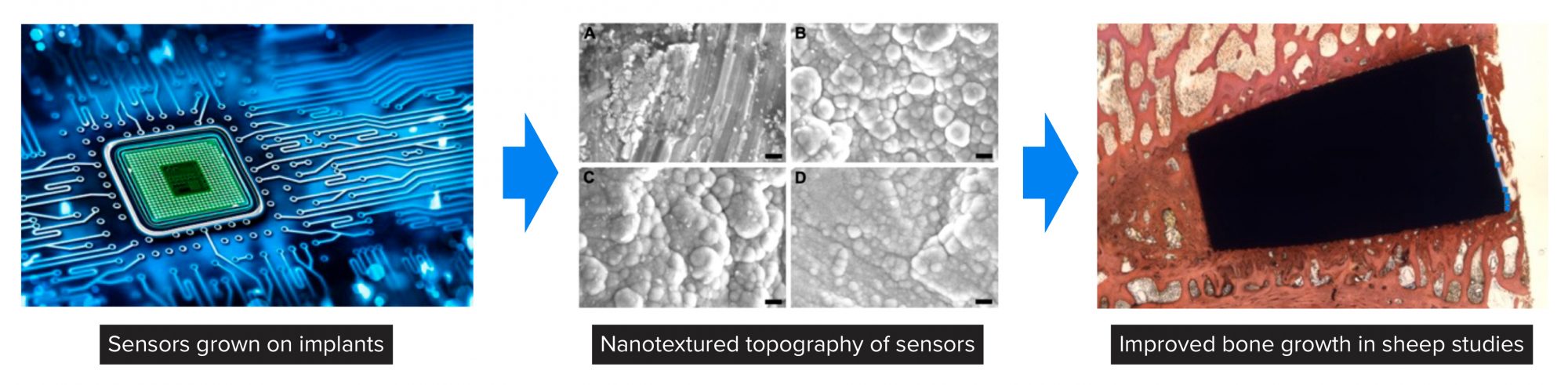
Lastly, Professor Webster is also involved in a company called Truss Health which is using AI and digital health to gather thermal scans from patients after implant surgery to predict implant success or failure. Shown in Figure 5 are thermal scans from a patient who recently received a knee implant.
Thermal scans were used to assess whether infection and/or chronic inflammation are setting in since both implant failure modes will increase local temperature. Such approaches can also be helpful to assess when staples should be removed as the temperature profile should return to normal, indicating healthy tissue.
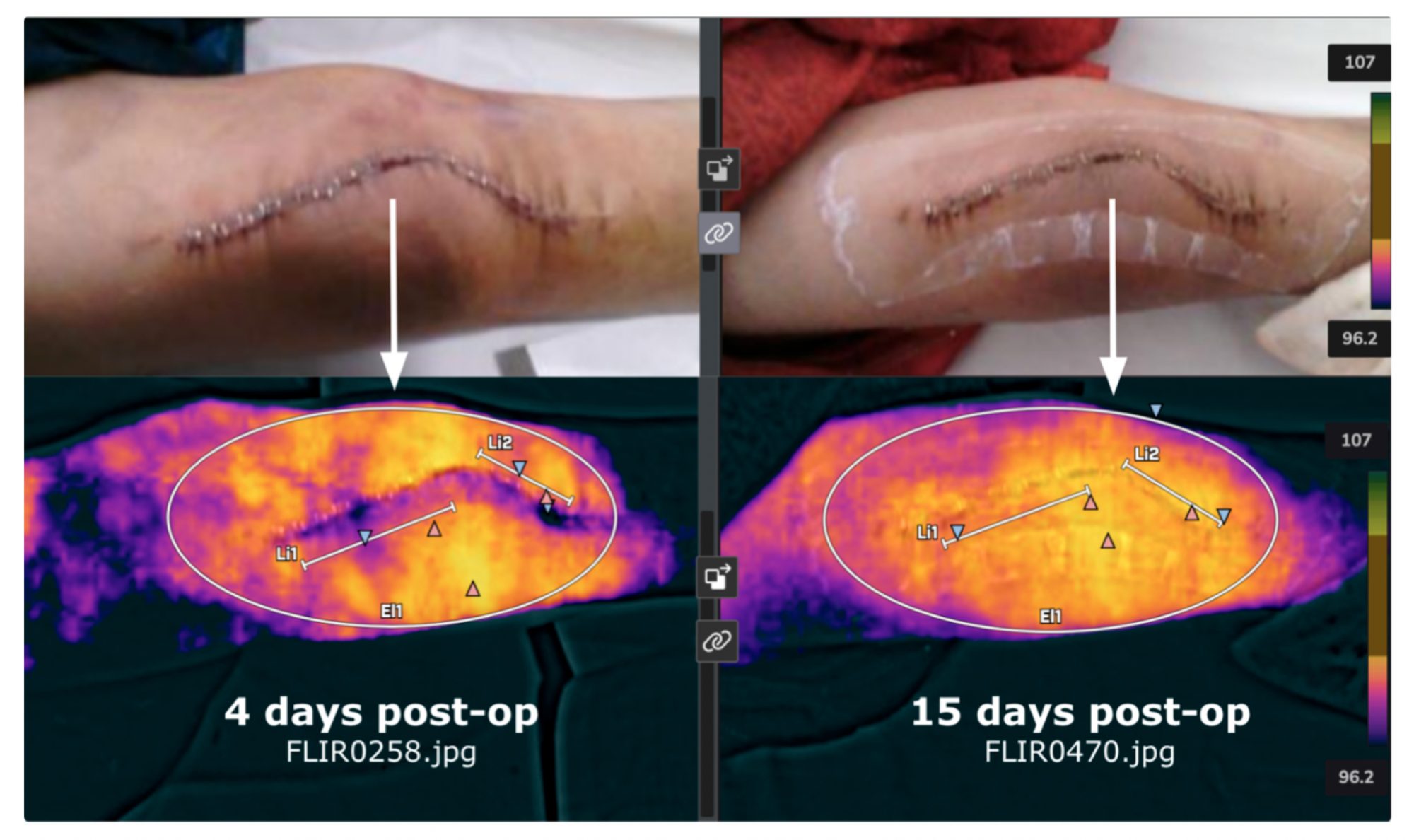
Summary
In summary, the four brief examples provided above demonstrate the promise of AI in medicine. Whether AI is being used to assess and predict disease treatment from a breathalyser, develop nanotextured surfaces personalised for that patient to ensure implant success, interpret implantable sensor function to predict implant outcomes, or assess thermal scans to assist in implant diagnosis, AI has a bright future in medicine. It just might be the BandAId™ healthcare needs to finally move beyond using a hip implant originally designed in 1962.
References
- https://www.merriam-webster.com/dictionary/artificial%20intelligence, accessed Sept. 8, 2023
- https://www.economist.com/special-report/2018/03/28/the-sunny-and-the-dark-side-of-ai, accessed Sept. 8, 2023
- https://spectrum.ieee.org/self-driving-cars-2662494269, accessed Sept. 8, 2023
- Gomez PF and Morcuende JA, Iowa Orthop J. 2005; 25: 30–37
- https://boydbiomedical.com/articles/the-top-7-reasons-why-medical-devices-fail#:~:text=Medical%20device%20startup%20owners%20fail,test%2C%20and%20market%20their%20devices, accessed Sept. 8, 2023
- https://www.cdc.gov/nchs/pressroom/nchs_press_releases/2022/20220831.htm#:~:text=Life%20expectancy%20at%20birth%20in,its%20lowest%20level%20since%201996,accessed Sept. 8, 2023

This work is licensed under Creative Commons Attribution-NonCommercial-NoDerivatives 4.0 International.