Photosynthesis serves as the primary mechanism for converting solar energy into chemical energy and plays a pivotal role in regulating atmospheric oxygen levels and carbon dioxide concentrations, influencing global climate patterns as a result
Traditional photosynthetic pigments, such as chlorophyll, can only absorb specific regions of the electromagnetic spectrum, leaving a significant span of wavelengths of light unutilized. The solar energy-conversion efficiency of photosynthesis typically reaches a maximum of ~6%; however, some plants exhibit efficiencies lower than 1%, depending on the type of organism, environmental conditions, and the specific metabolic pathway involved. While photosynthesis has relatively low efficiency compared to some human-made solar energy technologies, it plays a crucial role in sustaining life on Earth by providing the primary source of energy for most ecosystems.
The inefficiency of photosynthesis poses a significant challenge, especially in the context of addressing the climate crisis and the urgent need for sustainable energy sources. Supercharging photosynthesis, or enhancing its efficiency, is of vital importance in addressing global challenges such as food security and renewable energy production. One promising avenue for improving photosynthetic efficiency involves leveraging semiconductor nanoparticles, such as quantum dots (QDs), to enhance light harvesting. Nanoparticles can absorb a wider range of light wavelengths and channel electrons to nearby organisms, as well as alter the surrounding environment of the organism, enhancing conditions conducive to efficient photosynthesis.
The integration of semiconductor nanoparticles into organisms shows promise for boosting energy conversion efficiency and opening up new applications in biotechnology and beyond.
Photobiohybrids
Biohybrids are organisms, systems, or materials that combine biological components with inorganic materials or technologies for enhanced functionality or performance. Photosynthetic biohybrids, or “photobiohybrids,” refers to a subtype of biohybrid that integrates biological components with inorganic materials to enhance light harvesting and energy conversion capabilities. Photobiohybrid could also refer to materials, systems, or organisms. In material science, photobiohybrids include bio-solar cells using chloroplasts isolated from plants. In integrated systems, combining biological and synthetic components has wide applications, including environmental remediation.
This eBook will focus on photobiohybrid organisms, specifically bacteria, that enhance light harvesting capabilities by interacting with quantum dots. These nanoparticles absorb light and transfer electrons to the bacterial cell, where they can be utilized in various biochemical processes. This process has been observed in non-photosynthetic bacteria as well as across photosynthetic organisms, including plants, algae, and bacteria.
Bacterial photobiohybrids, also known as semiconductor-bacteria hybrids, are bacteria that decorate themselves with QDs that efficiently harvest light energy, which the cell can use to create valuable chemicals, fuel, and plastics. Essentially, the bacteria are uptaking or using inorganic materials around them to synthesize and cover themselves in tiny solar panels. This provides energy and a protective “shell,” helping them improve photosynthesis and survive in places where they couldn’t make it on their own. (Sakimoto K. et. al) Biomineralization is a fascinating natural process through which living organisms can synthesize and manipulate inorganic minerals. In the context of photosynthetic quantum dots, biomineralization involves the enzyme-facilitated synthesis and assembly of semiconductor nanocrystals, such as cadmium sulfide (CdS) or lead sulfide (PbS). Biomineralization pathways enable precise control over the size, shape, and composition of the quantum dots, resulting in uniform and well-defined nanocrystals with tailored optical and electronic properties. In addition to nanoparticle synthesis, some bacteria can facilitate nanoparticle uptake.
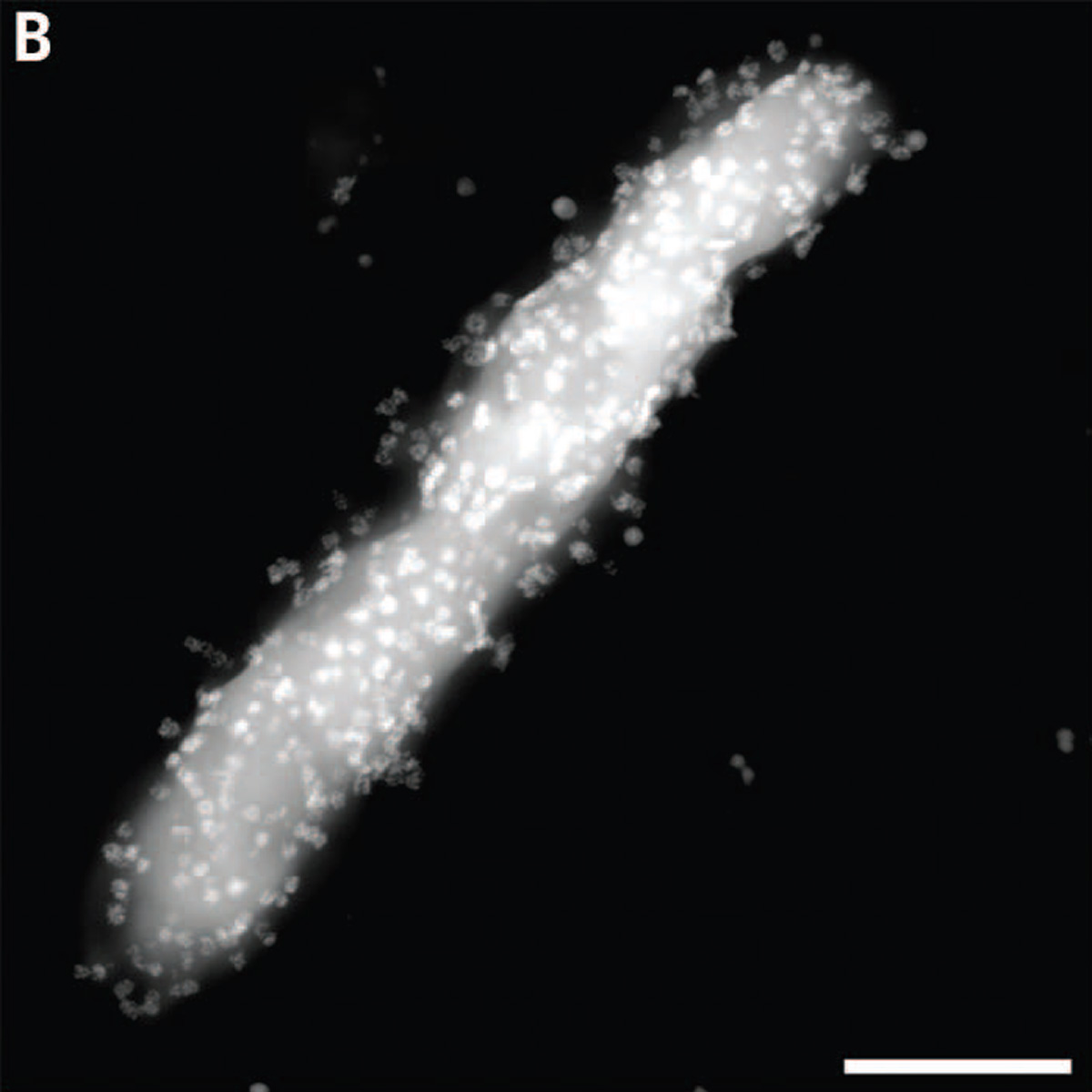
When semiconductor nanoparticles, like quantum dots, absorb photons of light, they can excite electrons, which can transfer to the bacterial cell and enter the electron transport chain, providing the cell with energy for various biochemical processes. When electrons are excited from the valence band to the conduction band, a “hole” is left behind. To maintain charge balance within the quantum dot, a sacrificial electron donor can fill the hole left in the valence band. Commonly cysteine, a sulfur-containing amino acid, is used as a reducing agent in photobiohybrid experiments, providing an electron to fill the hole, thus stabilizing the quantum dot and facilitating continuous electron transfer from the quantum dot to the bacterial cell. Figure 2 illustrates how a sulfur atom, such as from cysteine, donates the electron.
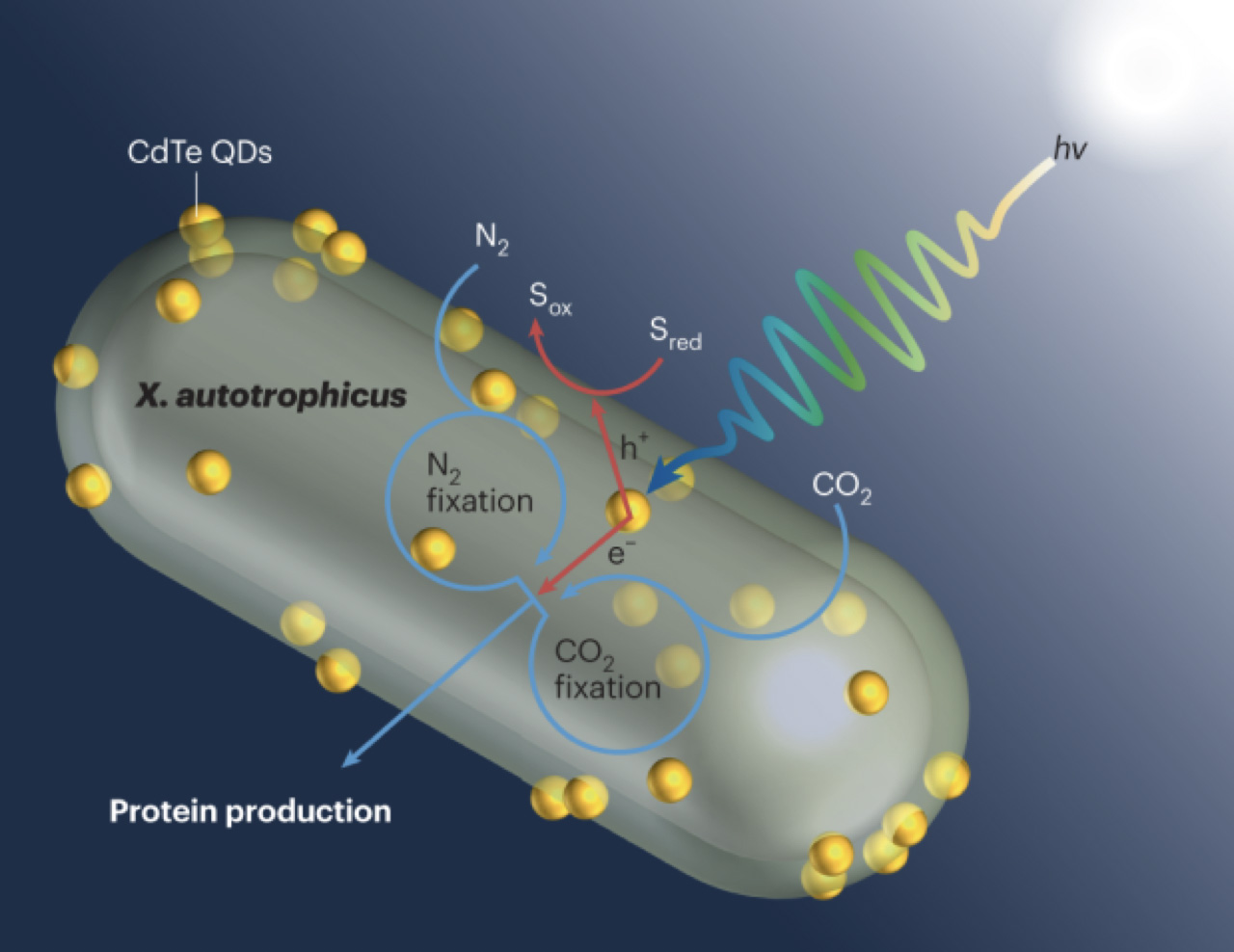
Figure 2. Both Figure 2A and Figure 2B depict X. autotrophicus-CdTe hybrids using QDs for CO2 and N2 fixation. Figure 2B expands on the overview provided in Figure 2A, highlighting the cellular structures involved.
Quantum dots are incredibly efficient at capturing light energy, which can be transferred to bacteria and used to produce valuable chemicals, fuel, and plastics.
The solar energy-conversion efficiency of quantum dots varies, but theoretical calculations suggest it can be as high as 70-90%, significantly outperforming natural photosynthesis and solar panels.(Geyer et. al) A study involving a single species each of microalgae, bacteria, and cyanobacteria revealed a substantial improvement in photosynthetic productivity, up to 2.5 times, by using QDs to upconvert underutilized wavelengths of sunlight. (Murray A. et. al)
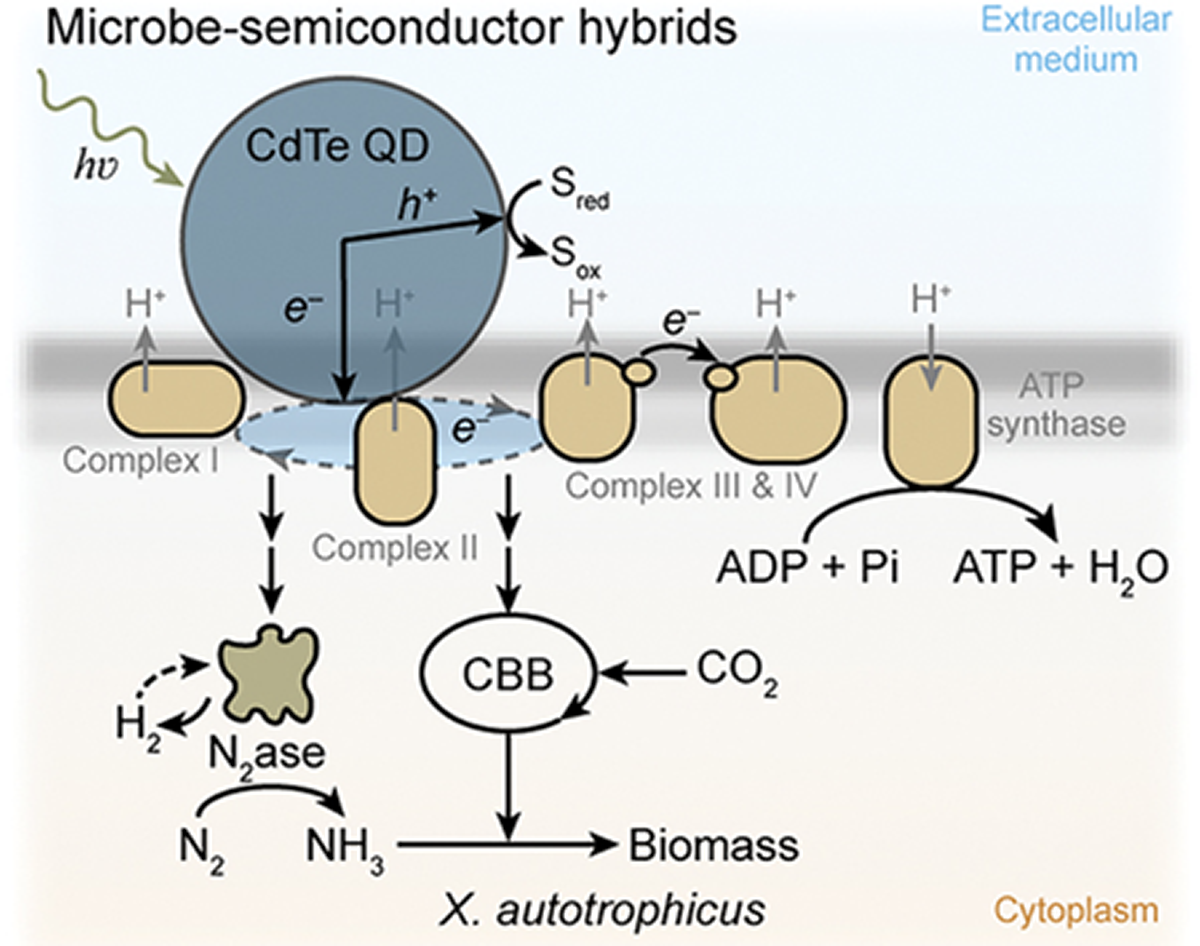
The integration of semiconductor nanoparticles into organisms shows promise for boosting energy conversion efficiency and opening up new applications in biotechnology and beyond. This process capitalizes on nanoparticles’ unique properties to enhance functionality and performance. This process has been observed in non-photosynthetic bacteria as well as across photosynthetic organisms, including plants, algae, and bacteria.
Harnessing QDs to enable heterotrophic bacteria and non-photosynthetic autotrophs to photosynthesize
Heterotrophic organisms, reliant on organic carbon sources for energy and growth, and non-photosynthetic autotrophs, reliant on chemosynthesis or other external energy sources, face inherent limitations in environments with limited access to such resources. These constraints often restrict their metabolic capabilities and hinder their ability to thrive in diverse ecological niches. However, the integration of quantum dots presents a revolutionary solution to overcome these limitations. By synthesizing or being equipped with QDs, heterotrophs and other non-photosynthetic organisms gain the unprecedented ability to harness light energy, an abundant and accessible energy source.
This transformative technology not only expands the metabolic capacity of non-photosynthetic organisms but also enables them to produce valuable compounds using sunlight, carbon dioxide, and water. In this way, QDs have the potential to revolutionize the role of heterotrophs in bioproduction, environmental remediation, and sustainable agriculture, offering unprecedented opportunities for addressing global challenges in food security, renewable energy, and environmental sustainability. Some examples include non-photosynthetic autotrophic bacterium Xanthobacter autotrophicus and heterotrophic bacterium Moorella thermoacetica.
QDs’ use of “Waste Light” in photosynthetic bacteria
Building upon the success of using QDs to enable heterotrophic bacteria to photosynthesize, researchers have also explored the potential of leveraging QDs to enhance photosynthesis in naturally photosynthetic organisms, such as plants, algae, and cyanobacteria. By incorporating QDs into these organisms’ photosynthetic systems, researchers aim to optimize energy conversion efficiency and mitigate the limitations of traditional photosynthetic pigments.
One particularly exciting aspect of QDs’ application in photosynthetic bacteria is their ability to harness “waste light” – wavelengths of light that are typically underutilized by natural photosynthetic pigments. Quantum dots can capture a broader spectrum of light, including wavelengths that traditional pigments struggle to absorb efficiently. By converting this waste light into usable energy, QDs effectively enhance the overall photosynthetic efficiency of the organism.
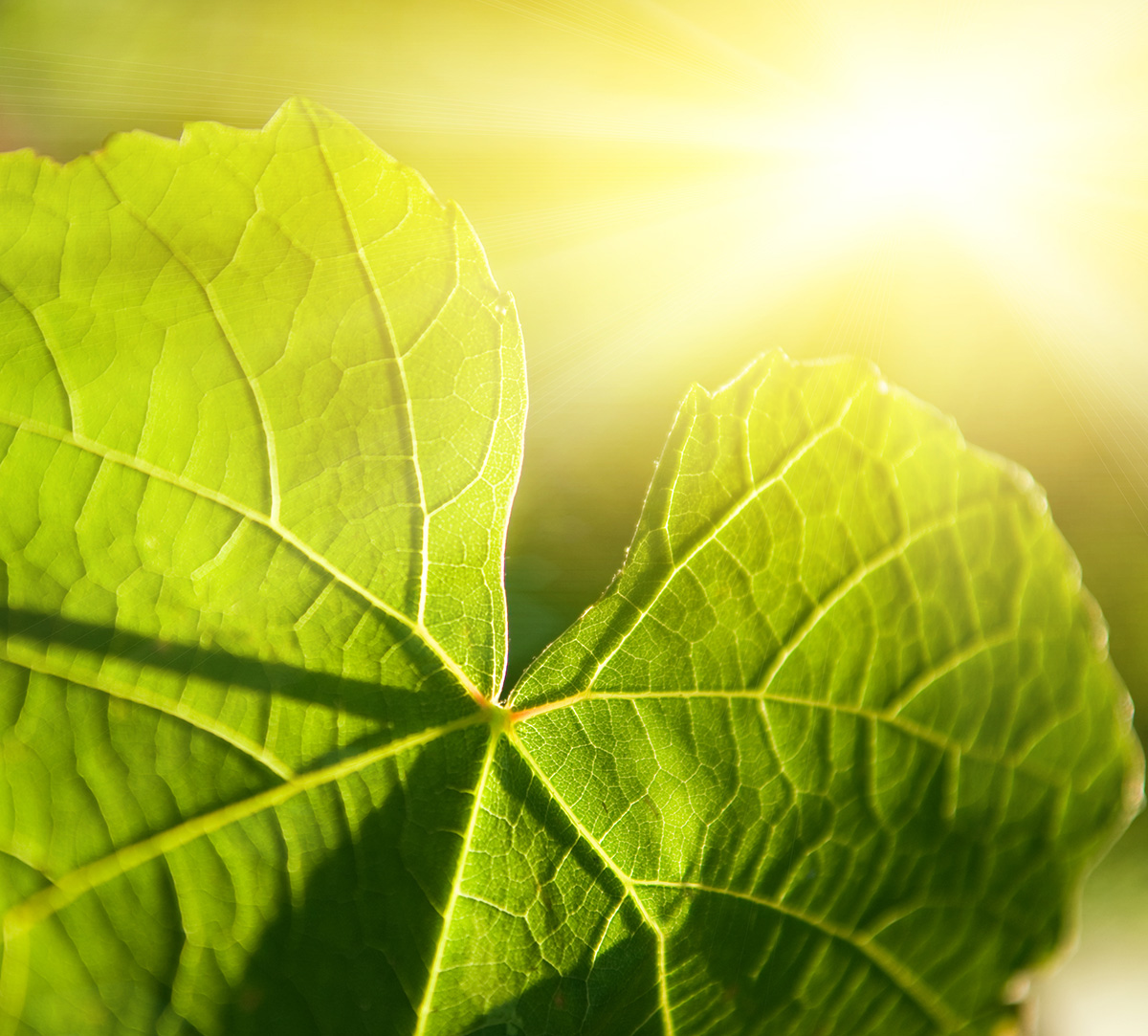
Additionally, the integration of QDs into photosynthetic bacteria offers a promising avenue for addressing environmental challenges, such as fluctuating light conditions and nutrient availability. By supplementing natural photosynthetic pathways with QD-mediated light harvesting, organisms, such as Rhodopseudomonas palustris, can maintain optimal photosynthetic activity under varying environmental conditions, leading to improved growth rates and biomass production.
Overall, the utilization of QDs to exploit waste light in photosynthetic bacteria represents a significant advancement in biotechnology, with far-reaching implications for sustainable energy production and ecosystem resilience. Researchers continue to unravel the intricacies of QD-mediated photosynthesis, paving the way for sustainable solutions to global challenges in food security, renewable energy, and climate change mitigation.
Harnessing the power of quantum dots
Photosynthesis stands as a cornerstone of life on Earth, enabling the conversion of sunlight into chemical energy crucial for sustaining ecosystems and supporting life. However, traditional photosynthesis exhibits inherent inefficiencies, limiting its potential to address pressing global challenges such as climate change, food security, and sustainable energy production. The integration of biology with semiconductor nanoparticles, particularly quantum dots (QDs), has emerged as a transformative strategy to enhance photosynthetic efficiency and unlock new avenues for innovation in biotechnology. By leveraging the unique properties of QDs, researchers have revolutionized the photosynthetic capabilities of both photosynthetic and non-photosynthetic organisms.
While much is still unknown about the metabolic pathways and intricacies of QD-mediated photosynthesis, it is evident that harnessing the power of quantum dots holds the potential to revolutionize our approach to energy production and environmental conservation, paving the way for a brighter tomorrow.