Open Access Government interviews Dr Carlos Ziebert, of the Karlsruhe Institute of Technology (KIT), who explores the thermal and safety properties of batteries across calorimetric studies
Could you briefly summarise your work and your current projects?
The group batteries – calorimetry and safety – focus on calorimetric studies and safety tests on lithium-ion cells and post-lithium cells. Depending on the cell size and application, different types of calorimeters are used in Europe’s largest Battery Calorimeter Laboratory, established in 2011. It provides seven Accelerating Rate Calorimeters (ARCs) from Thermal Hazard Technology, allowing the evaluation of thermodynamic, thermal and safety data for Lithium-ion and post-Li cells on material, cell, and pack levels for both normal and abuse conditions (thermal, electrical, mechanical). The lab also includes glove boxes for cell assembly and disassembly, many temperature chambers, a thermal camera, and cyclers with several hundred channels. It contains extremely sensitive 3D Calvet calorimeters, providing thermodynamic parameters and gas chromatography-mass spectrometry systems from Perkin-Elmer for venting gas analysis.
The group’s work can be categorized into the following six areas
- Small cell assembly and thermal studies of cell components
- Ageing tests
- Heat generation under normal use
- Heat, gas and pressure generation under thermal, electrical, and mechanical abuse
- Thermal propagation tests on small pack level
- Electrochemical impedance spectroscopy and modelling
The group is interdisciplinary and well-connected across both Germany and Europe and pursues a comprehensive approach that ranges from basic studies to industrial applications. Starting with four members in February 2020 the group has been growing up to fifteen members due to the successful funding of two national projects, which are called AnaLiBa and BatgasMod and a European Horizon 2020 project named HELIOS.
A) AnaLiBa
The AnaLiBa project (the Analytics of Lithium-Ion-Batteries), funded with €1.3 million, aims for the joint method development of a standardised sample-handling process for the gas analysis of large cells. This encompasses a method in which a pressure-tight canister is connected via a small capillary to a gas mouse and the direct insertion of the capillary into the cell. Finally, it provides a direct coupling of the ARC and an online mass spectrometer. Knowledge of the gas composition under the various cell abuse scenarios reveals the relevant decomposition paths and gives an idea, of which protective measures are necessary, for example, to delay or prevent the cell fire caused by the ignition of vent gases or thermal runaway. In the project both different cell formats, different cell capacities and different areas of application (high-energy/high-performance cells) will be compared.
B) BatgasMod
The second national project is called BatgasMod (Modelling of Battery Gasing) [2], which began on the first of October 2021. It belongs to the new battery competence cluster Battery usage concepts (BattUse). This competence cluster BattUse aims to foster our understanding of battery behaviour to determine when the second use of battery storage is preferable, funded by the German Federal Ministry of Education and Research – BMBF by €20 million.
The reactions of the liquid electrolyte and the associated gas formation play a decisive role in cell ageing and cell safety. These are, therefore the focus of the BatgasMod project funded by the BMBF with around 1.3 million euros.
It is led by the IAM-AWP at KIT, other partners are the Institute for Power Electronics and Electrical Drives (ISEA) at RWTH Aachen University and the Münster Electrochemical Energy Technology (MEET) at the University of Münster. The aim of the project is to develop electrolyte ageing models in combination with battery models for the early prediction of cell behaviour in the usage phase.
C) HELIOS
In the European project HELIOS (High-pErformance moduLar battery packs for sustaInable urban electrOmobility Services), achieving €10 million in funding since January 1st 2021, improved modular battery packs are being developed together with 18 partners from Europe and Turkey under the lead of Aarhus university.
Applying a holistic circular approach, the HELIOS project investigates optimal eco-designs and advanced processes to both enhance and demonstrate innovative, lighter, and eco-friendly hybrid Li-Ion-based battery packs for mid-size vehicles and city buses.
How does your work ensure lithium-ion batteries’ safety and high performance regarding cell ageing?
Safety issues have a major influence on consumers’ willingness to adopt the current Li-ion battery technology, due to an uncontrollable temperature increase (so-called thermal runaway) can cause an ignition or even explosion of the battery with the simultaneous release of toxic gases. Thus, thermal management and safety are of utmost importance for the electrification of transport and for stationary storage.
The abuse or safety testing has the main objective to identify all possible risk conditions to clearly define mitigation measures to be used in the design, control and usage of the cells and packs. It is carried out using seven ARCs of different sizes – from cylindrical to large pouch or prismatic automotive format – by applying electrical, mechanical, and thermal abuse scenarios and studying the heat, gas and pressure generation under these conditions. This allows quantifying critical parameters and their thresholds for safe cell operation.
The influence of ageing on the hazard potential can be quantitatively determined by performing three types of safety tests in the ARCs
- Electrical abuse: External/ internal short circuit test, overcharge test, over-discharge test
- Mechanical abuse: Nail test
- Thermal abuse: Heat-Wait-Seek test, ramp heating test, thermal propagation test
Self-heating, thermal stability and thermal runaway are characterized and the critical parameters and their thresholds for safe cell operation are determined. Another important issue are the venting gases, which can be collected during the abuse tests and analysed ex-situ or measured in operando using an online mass spectrometer. For the ex-situ method, a gas chromatography (GC) method with two detectors (mass spectrometry (MS) and thermal conductivity detector (TCD)) are available to detect and quantify the gases. Knowledge of the gas composition under the various cell abuse scenarios reveals the relevant decomposition paths and gives an idea of which protective measures are necessary, e.g., to delay or prevent the cell fire caused by the ignition of vent gases or thermal runaway.
If it is not possible to stop a single cell from going into thermal runaway, the propagation of the thermal runaway to the neighbouring cells, which is called thermal propagation, must be prevented or at least extended by 5-10 min. The largest ARCs allow studying the thermal propagation on a small pack level to develop and qualify suitable heat protection barriers, which give the passengers in an electric vehicle enough time to escape or to be rescued by the firefighters.
The cycle life can be studied by performing cyclic ageing tests and characterising the cells at fixed time intervals with respect to changes in heat generation and temperature profile, which serves as a “fingerprint” for the state-of-health (SOH). Comprehensive ageing tests are carried out at the cell level for all cell sizes.
At first, fresh cells are stored in temperature chambers at different temperatures and states of charge and then characterized at fixed time intervals to study the influence of this storage (calendar ageing) on cell performance. Second, cells of the same type are aged at different charge/discharge rates or with different load profiles (full cycles, partial cycles, driving cycles, stationary storage cycles (cyclic ageing). Once the cells have reached their termination criterion, the reversible and the irreversible heat are measured using entropy measurement and electrochemical impedance spectroscopy (EIS). These data can be used for the parameterization of impedance-based electrical equivalent circuit models (EECMs) and ageing models. In addition, some cells are analysed post-mortem – i.e. disassembled in an inert gas atmosphere and the changes in the chemical composition – the morphology and the structure of the electrodes are determined.
Battery calorimetry provides quantitative and system-relevant data for temperature, heat and pressure development of materials and cells as fast feedback for cell development and as input data for simulations.
How can we reduce the degradation of the LIB?
To guarantee a permanently high performance of lithium-ion batteries (LIB) in long-term operation requires the perfect interaction of the constituent components, such as electrodes, electrolytes, separators and current collectors. However, extensive material reactions take place during permanent charging and discharging, but also during the initial battery formation, which can adversely affect the behaviour of the LIB. It is not possible to completely avoid this degradation of the LIB, but an improved understanding of the different ageing processes helps to reduce the degradation.
The work at KIT focuses on two areas
Extensive battery calorimetric measurements are carried out on the active materials and the electrolytes as well as on the cells for consistent modelling to better understand the changes and to reveal the decomposition mechanisms of the electrolyte and the simultaneous build-up of gas pressure during cell use. However, the results of the calorimetric measurements are included in the thermodynamic modelling of the electrolyte components, the additives, and the electrode materials with the help of CALPHAD (CALculation of PHAse Diagrams) methods, which are used to calculate phase diagrams and thermodynamic functions that are implemented in the overall model.
The overall model makes it possible to understand the decomposition of the battery electrolyte during battery use and the effects on ageing. Therefore, safety can be increased, and the LIB can be operated much longer without disruption, which increases its sustainability. By transferring the model to newly developed materials and cells, their time-to-market is shortened, which allows the model to check performance and service life regarding electrolyte ageing at an early stage. This serves both the mission of the BattUse cluster and the innovation pipeline of the BMBF umbrella concept for the German battery research factory.
How do the HELIOS battery packs guarantee sustainability, performance and improved safety?
HELIOS believes that a new concept of a standardised, modular, and scalable battery pack is possible and that it will be efficient for use in a varied range of applications. Capitalising on advanced materials and top-notch modelling Big Data and Information and Communication Technologies (ICTs), the new battery pack is expected to combine improved autonomy and charging performance with enhanced safety standards and a minimum carbon footprint.
By bringing together the expertise of industry and academia, the HELIOS project aims at developing and integrating innovative materials, designs, technologies, and processes to create a new concept of a lighter, smarter, and more eco-friendly hybrid battery pack. The hybridization concept consists of a combination of high-energy (HE) and high-power cells (HP). While the HE cells ensure a long driving range, the HP cells should enable fast charging and additional power for a short period if needed. The final battery pack should be modular and scalable for a wide range of electric vehicles used in urban electromobility services and should provide improved performance, energy density, safety, lifetime, and LCoS (Levelised Cost of Storage). The range encompasses mid-size electric vehicles to electric buses. A Mitsubishi iMiEV at the Aarhus University and a Sileo S12 e-bus from Bozankaya will be used as demonstrators for the performance and improvements of the HELIOS modular battery packs.
For this purpose, novel developments that integrate hardware and software solutions for the smart control of electrical and thermal management systems that exploit advanced materials, power electronics, sensors, and cutting-edge ICT, such as cloud-based Big Data Analysis, Artificial Intelligence, and IoT (Internet of Things) technologies running in the cloud are investigated and implemented.
These combined approaches allow us to increase energy and power density and safety; enhance key characteristics like ultra-high power charging; improve E-fleet control and health management strategies to extend lifetime; create optimised EV charge; monitor the SOC (State of Charge), SOH (State of Health), and carbon footprint for each battery pack throughout its entire life cycle.
It also shows better LCA (life cycle assessment, new methodologies for recycling and metal recovery after EoL (end of life), and already a material selection with better overall LCA; improves battery pack design and performance with reduced LCoS based on a circular economy approach where the modular battery packs can be easily re-used in a range of second-life applications prior to EoL recycling; and assesses the HELIOS solution effectiveness in different urban electromobility models.
I lead Work Package 4 – ‘Cell and battery pack testing and modelling’, whose main objectives are to expand and improve the state-of-the-art within cell and battery modelling, state estimation, fault detection, prognostics, and health management by utilizing the available information from various sensing technologies and cell tests.
It is also expected that the battery pack can be safer by being able to detect internal faults inside the battery before the faults propagate. The main scientific objective of the work performed at KIT, DTI, and ZSW is to determine the coupled electrochemically, thermal, and safety data of both the HE and HP cells that are required as input parameters for the modelling or validation data for the simulation results. These data are acquired on the materials, cells, and pack level under both normal use and abuse testing scenarios for fresh and aged cells and are stored and aggregated in a data warehouse platform that has been provided by DTI. The extensive cell testing will generate a large amount of data that will help to improve the different levels of modelling that will be developed in the third year to bring the HELIOS project to the road to success.
References
- “Battery venting gas analysis using battery calorimeters & gas chromatography”, Open Access Government 33, pages 384-385.
- “Correlation of electrolyte properties, gas formation and heat generation by electrochemical-calorimetric methods”, Open Access Government 35, pages 382-383.
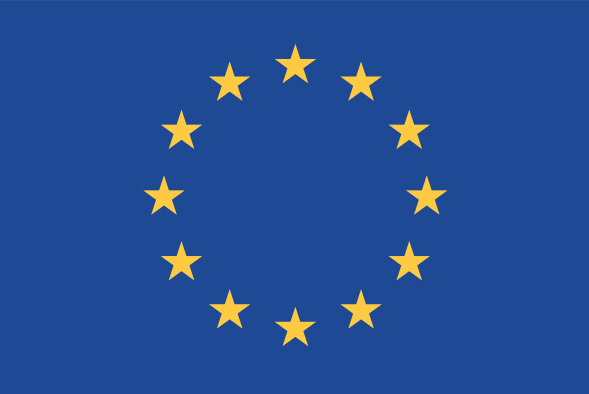
This document reflects the view of its author only. The funding agency is not responsible for any use that may be made of the information it contains.

This work is licensed under Creative Commons Attribution-NonCommercial-NoDerivatives 4.0 International.