Christopher H. Contag from the Institute for Quantitative Health Science and Engineering (IQ) at Michigan State University discusses the potential of engineered endosymbionts as biologically encoded remote controls for regenerative medicine
Taxonomic definitions divide all forms of life on Earth into three domains called Archaea, Bacteria, and Eukarya; these domains of life are distinguishable by cellular structures, processes of cell division, and molecular signatures. (1) About 2.2 billion years ago, the Eukarya domain of life began from interactions between two single-celled organisms, one from the domain Bacteria that was living inside a cell from the domain Archaea.
Over billions of years, the two cells co-evolved, with the internal cell becoming the mitochondria of the outer cell. This theory on the origin of Eukaryotes, those forms of life in the domain Eukarya, is called the endosymbiont theory (the prefix endo meaning inside) and was first proposed by a Russian botanist Konstantin Mereschkowski in 1910 and then further substantiated by Lynn Margulis (previously Lynn Sagan) in 1967. (2)
The process of creating endosymbionts, called endosymbiogenesis, occurs naturally in a wide variety of species (for example in insects). (3,4)
Recently, it has been demonstrated that endosymbiogenesis can be re-created in the laboratory by modifying otherwise free-living bacteria into endosymbionts that can survive inside and interact, with yeast (5,6) or, as we have shown, as functionally engineered endosymbionts in mammalian cells (Fig.1). (7)
We began engineering endosymbionts to create biological remote control modules that we can use to direct the fates and functions of mammalian cells from a distance. (7) Much like a TV remote control can be used to change the channels and settings of your television, engineered endosymbionts could be used to change the settings and functions of cells in the body. This way, it may be possible to control cells in the liver, heart, brain, or other tissues using energy from outside the body. For example, engineered endosymbionts could control immune cells (7) or be used to guide tissue regeneration and deployed in the body to replace lost or damaged tissues. In the initial studies, we used sugars to control the engineered endosymbionts. (7)
We have also been investigating ways to both image and control endosymbionts from outside the body with electromagnetic energy. (8,9,10)
To demonstrate the possibility of external control of mammalian biology, we have engineered bacterial cells to respond to alternating magnetic fields by locally generating heat that can, in turn, activate genetic programs using tunable thermal switches. (9,11) Since magnetic fields can be delivered anywhere in the body, it will be possible to generate local magnetothermal energy in specific organs to turn on or off genetic networks at will. Although not in the form of endosymbionts, bacteria have been used to redirect stem cells into heart cells by delivering proteins. These transcription factors activate genetic pathways in mammalian cells, suggesting that engineered endosymbionts could also be used to guide the regeneration of mammalian organs.
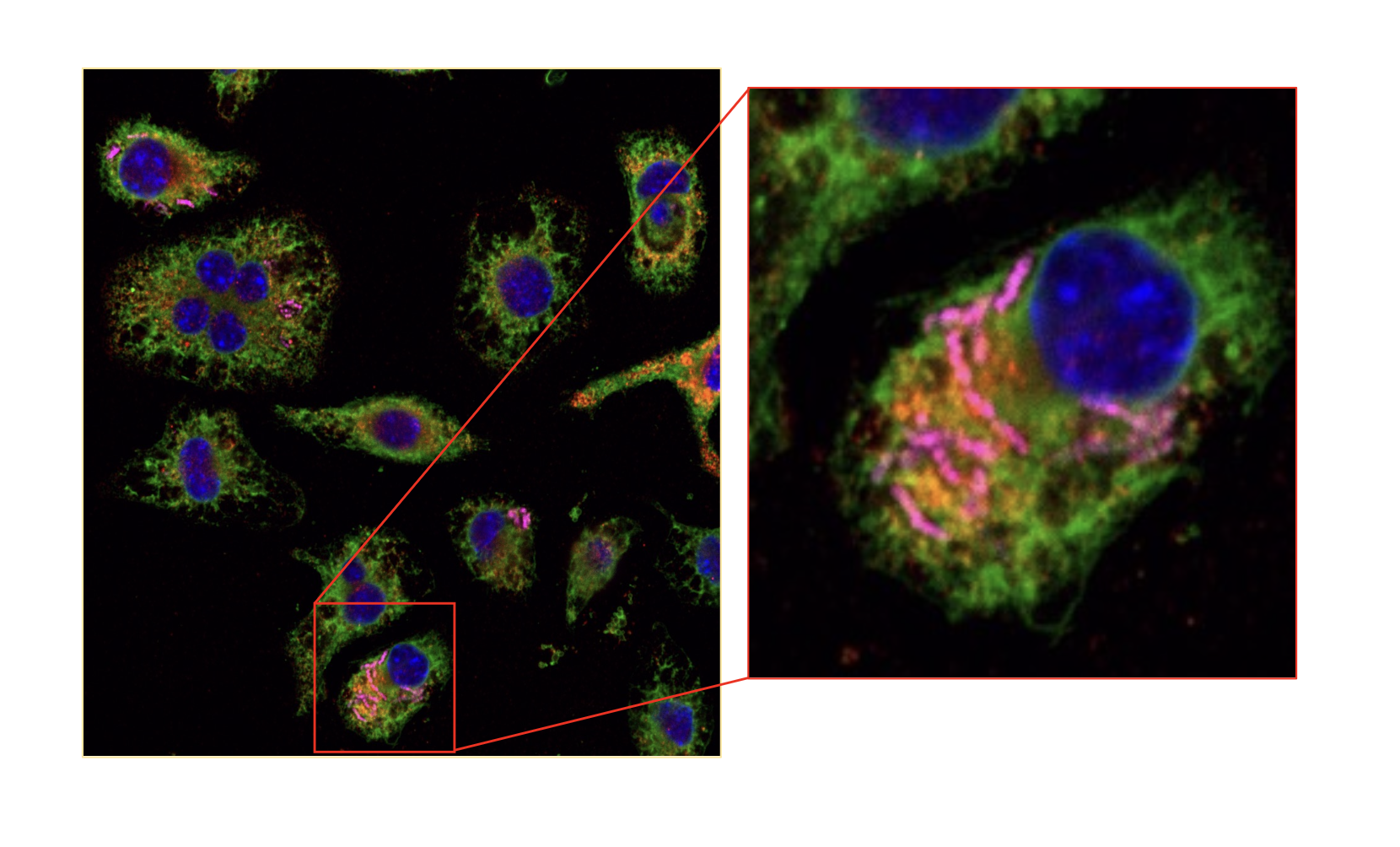
Lessons from nature
Endosymbionts are, by definition, cells of two different forms of life, one living inside the cell of the other (intracellular), and these symbiotic relationships occur naturally in many species. Intracellular bacteria can also have a pathogenic, disease-causing relationship with their hosts. It was likely the case that two billion years ago, the origins of the mitochondria and chloroplasts of plants resulted from a pathogenic interaction between different types of single-celled organisms that then evolved into a symbiotic relationship and eventually into organelles (mitochondria and chloroplasts) inside the host cells.
Organelles cannot live outside the host cell because they only have remnants of their original genomes as they evolved towards a minimal genome while relying on many functions from the host cell. The genes in these genome remnants have been used to infer the original species of Archaea. The naturally occurring interactions between bacteria and other cell types can teach us how to engineer endosymbionts, and the principles of synthetic biology can be applied to engineer endosymbiotic relationships. Synthetic biology is a field of technology that uses engineering principles in biological systems. In this way, parts of naturally occurring intracellular bacteria, such as Listeria monocytogenes, can be removed and used to convert other types of bacteria, such as Bacillus subtilis, into engineered endosymbionts. (8,12)
Regenerative medicine
The discovery of the proteins that can convert differentiated cells into pluripotent stem cells (13) revolutionized the field of regenerative medicine by enabling the generation of stem cells from adult cells, and these induced pluripotent stem cells (iPSC) could then be differentiated into any type of specialized cell. The transcription factors used to make iPSC, Oct4, Sox2, Klf4, and c-Myc, have been introduced in various ways into mammalian cells, including in human cells. (14) These transcription factors control multiple genetic networks that then reprogram cells. Many ways of introducing the genes that encode these factors into host cells result in genome modification, raising a regulatory challenge for medical applications. Engineered endosymbionts can deliver transcription factors to cells without modifying the host genome (8).
Engineered Endosymbionts can be engineered to deliver large amounts of genetic cargo that can control many cellular processes using a myriad of genetic controls and switches. These features make engineered endosymbionts a potentially powerful tool for cellular control. Stem cells have a dramatic replicative capacity, and a small number, even one (15) of these cells, can regenerate an entire tissue; this capability can compensate for the anticipated inefficiency of uptake of engineered endosymbionts by mammalian cells. The potential for guiding cellular fates and functions with engineered endosymbionts is extraordinary.
Minimal genomes
During evolution, the genomes of organelles were reduced to a minimum size to provide only limited functions, ensuring dependency on the functions from the host. Recently, a completely synthetic bacterial genome has been used to engineer a minimal genome bacterial cell, which is still capable of autonomous replication. (16)
The limited capability of this minimal genome bacterium offers an ideal chassis on which to build the next generation of engineered endosymbionts. We can use the lessons learned from both eukaryotic evolution and natural intracellular bacteria and the opportunities to engineer minimal genome bacteria to create engineered endosymbionts that function as transplantable, portable, synthetic organelles to control a wide range of cellular functions. The field of engineered endosymbionts is in its infancy, but the potential of this approach for engineering integrated biosystems and revolutionizing medicine is immense.
References
- Woese, C. R., Kandler, O. & Wheelis, M. L. Towards a natural system of organisms: proposal for the domains Archaea, Bacteria, and Eucarya. Proc Natl Acad Sci U S A 87, 4576-4579, doi:10.1073/pnas.87.12.4576 (1990).
- Sagan, L. On the origin of mitosing cells. J Theor Biol 14, 255-274, doi:10.1016/0022-5193(67)90079-3 (1967).
- Konecka, E. Fifty shades of bacterial endosymbionts and some of them remain a mystery: Wolbachia and Cardinium in oribatid mites (Acari: Oribatida). J Invertebr Pathol 189, 107733, doi:10.1016/j.jip.2022.107733 (2022).
- Kaur, R., Shropshire, J. D., Cross, K. L., Leigh, B., Mansueto, A. J., Stewart, V., Bordenstein, S. R. & Bordenstein, S. R. Living in the endosymbiotic world of Wolbachia: A centennial review. Cell Host Microbe 29, 879-893, doi:10.1016/j.chom.2021.03.006 (2021).
- Mehta, A. P., Supekova, L., Chen, J. H., Pestonjamasp, K., Webster, P., Ko, Y., Henderson, S. C., McDermott, G., Supek, F. & Schultz, P. G. Engineering yeast endosymbionts as a step toward the evolution of mitochondria. Proc Natl Acad Sci U S A 115, 11796- 11801, doi:10.1073/pnas.1813143115 (2018).
- Mehta, A. P., Ko, Y., Supekova, L., Pestonjamasp, K., Li, J. & Schultz, P. G. Toward a Synthetic Yeast Endosymbiont with a Minimal Genome. J Am Chem Soc 141, 13799-13802, doi:10.1021/jacs.9b08290 (2019).
- Madsen, C. S., Makela, A. V., Greeson, E. M., Hardy, J. W. & Contag, C. H. Engineered endosymbionts that alter mammalian cell surface marker, cytokine and chemokine expression. Commun Biol 5, 888, doi:10.1038/s42003- 022-03851-6 (2022).
- Makela, A. V., Schott, M. A., Madsen, C. S., Greeson, E. M. & Contag, C. H. Magnetic Particle Imaging of Magnetotactic Bacteria as Living Contrast Agents Is Improved by Altering Magnetosome Arrangement. Nano Lett 22, 4630-4639, doi:10.1021/acs.nanolett.1c05042 (2022).
- Greeson, E. M., Madsen, C. S., Makela, A. V. & Contag, C. H. Magnetothermal Control of Temperature-Sensitive Repressors in Superparamagnetic Iron Nanoparticle- Coated Bacillus subtilis. ACS Nano 16, 16699-16712, doi:10.1021/acsnano.2c06239 (2022).

This work is licensed under Creative Commons Attribution-NonCommercial-NoDerivatives 4.0 International.