Professor Michel Moisan and his team at Université de Montréal (UdeM) explore reliable, energy-efficient and multi-purpose plasmas with microwaves for research and technology
The ability to generate ionized gases (gaseous plasmas) under required operating conditions (shape and volume of the plasma, nature of the gas (including various mixtures), and pressure) is central to many aspects of science and industry. In that respect, of particular interest are low-temperature plasmas where the electrons have high energy while the ions remain slightly above room temperature, allowing, among other things, low-energy chemistry.
To achieve reproducible and low-contaminated (electrodeless) gaseous discharges, it is better to call on a high-frequency (HF) electric field: it initially accelerates a few electrons that are randomly present in the gas, which then go on to strip the outer electrons of atoms in a source gas, creating an ‘avalanche’ process, which culminates in a stationary fluid of electrons and ions – a plasma.
Professor Michel Moisan and his team at Université de Montréal (UdeM) explored the capabilities of various devices they patented (7) (e.g. surfatron, surfaguide: now in the public domain) that provide plasma torches or plasma columns simply and efficiently, using radiofrequency (RF: few MHz-100 MHz) or microwaves (above 100 MHz in the present case), both commonly designated as HF fields. These original devices launch an electromagnetic surface wave that propagates guided along the plasma jet surrounded by a gas (e.g. air) or along a plasma column filling a dielectric vessel (transparent to HF field) in which it is generated.
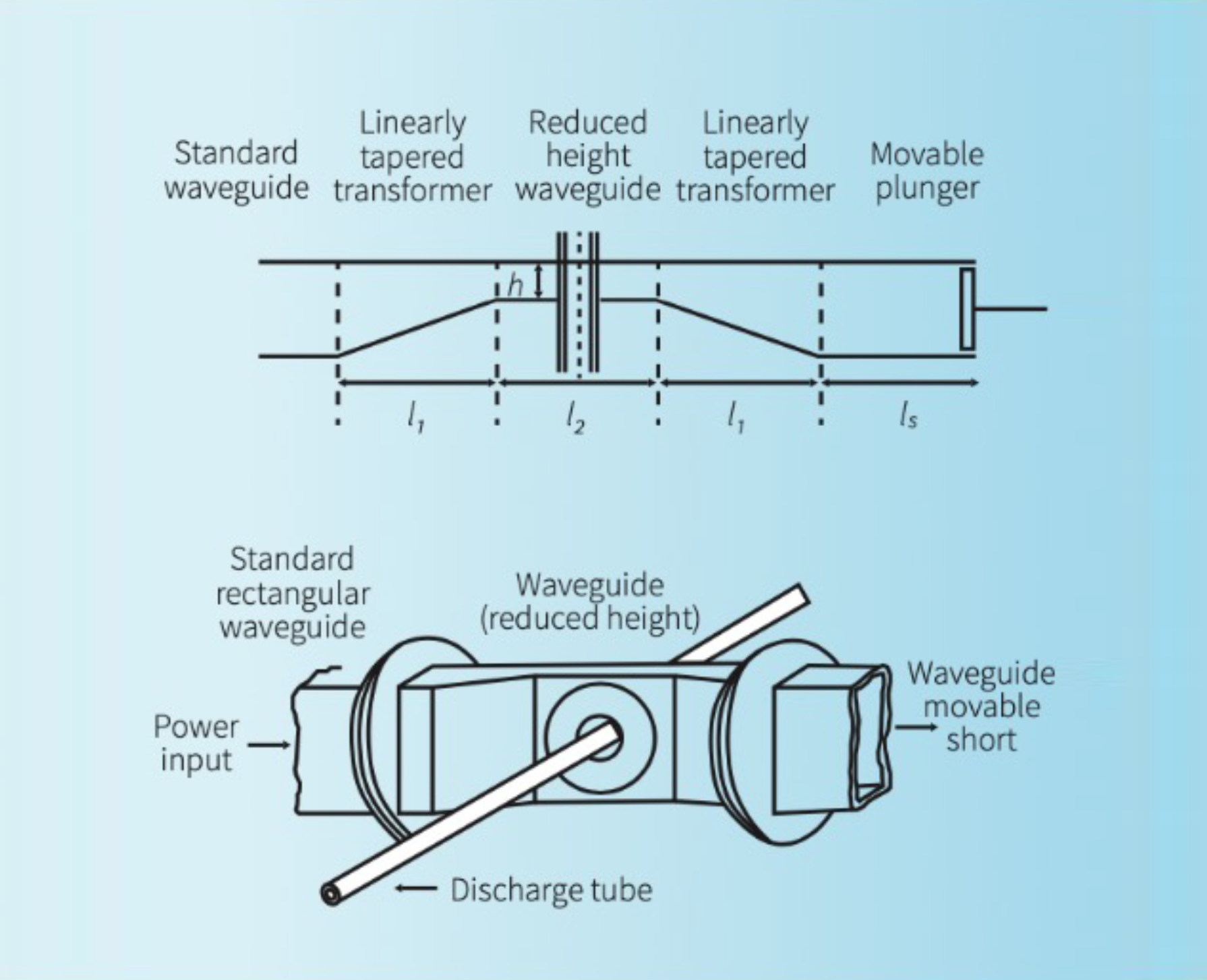
Understanding surface-wave (SW) sustained plasmas
“A critical comparison of the properties of RF and microwave (MW)-produced plasmas by different field applicators shows that the possibilities of the surface-wave-produced plasma in many ways exceed those of other existing RF and MW microwave-produced plasmas. In addition, since the plasma is sustained through wave propagation, the wave launcher can be small compared to the length of the plasma column. A further advantage of surface-wave-produced plasmas is that they can be obtained both in the RF and in the MW frequency domain” (1). It is thus possible to optimize the plasma parameters for a given application through varying the wave frequency since ions and electrons are entrained by RF fields whereas only electrons respond to MW fields.
The surfaguide: a power-efficient MW field applicator for plasma generation which is easy-to-build and operate
For plasma generation to work effectively, it is crucial for power to be transferred from the RF or MW generator to the plasma as efficiently as possible, while also ensuring that stable gas discharges can be sustained for extended periods of time.
In addition, this must be done across a broad range of operating conditions – including (abruptly) varying compositions and pressures in the gas. SW launchers (a family of HF field applicators) like the surfaguide (fig. 1) have been investigated and worked out such that they can be designed to withstand these types of changes, without running off (no impedance retuning with the HF power generator needed).
The plasma column is produced within a dielectric tube (e.g. fused silica (high-temperature discharge), AlN ceramic (resistant to fluorine)) and extends from both sides of the launching interstice. The surfaguide illustrated in the figure is based on a WR-340 rectangular waveguide, which ensures efficient operation at 2450 MHz, provided the discharge tube diameter does not exceed 15% of the waveguide wide wall area (here 12 mm) to operate on a large range of varying conditions and not needing retuning; at 915 MHz with a WR-975 frame (2), the tube diameter could reach up to 36 mm similarly. Continuous 6 kW operation at 2450 MHz is customary in the industry.
The waveguide height h is chosen such that the intrinsic impedance of the SW plasma column acting as a transmission line be close to the impedance of the surfaguide device at height h.
Some applications of surface-wave discharges (SWDs)
1. A compact cw chemical HF/DF laser with a SWD activated by a surfatron at 915 MHz
Fluorine atoms (from dissociated SF6 molecules) are mixed with hydrogen (or deuterium), and the chemical reactions which follow generate a large number of HF (or DF) molecules in excited vibrational states, creating a population inversion. Electrical efficiency calculated at the maximum laser output power is 0.6 % (at 600 W MW power, it makes 3.6 W: caution). From application 2, it was found better that the Al2O3 ceramic discharge tube be replaced by an AlN ceramic that resists fluorine corrosion and, additionally, that the input gas flow be vortexed to prevent the tube from breaking due to local overheating (5).
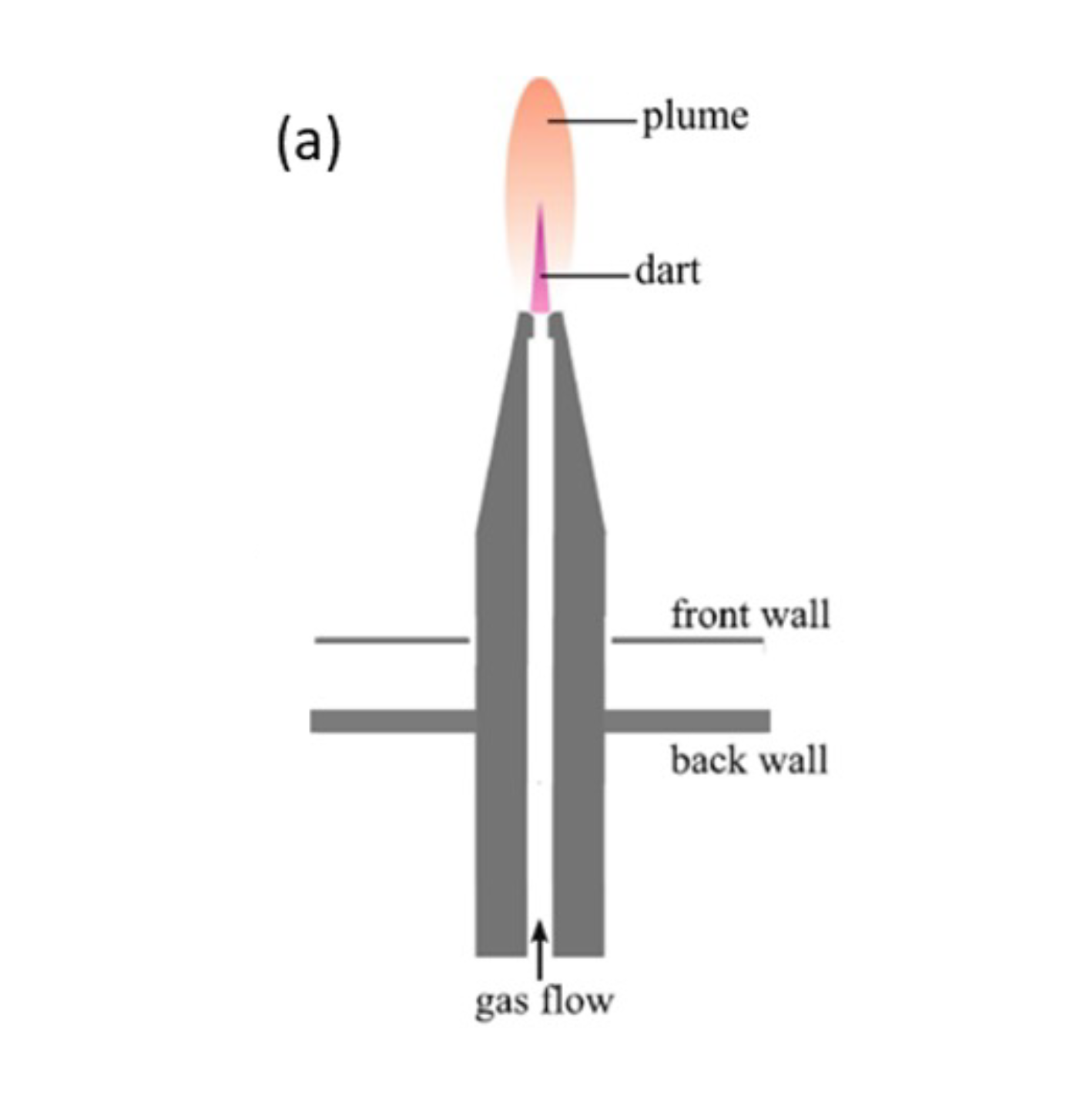
2. Eliminating greenhouse gases and purifying rare gases obtained from cryogenic air distillation
Surfaguide-based plasma systems were used to remove fluorinated greenhouse gases such as CF4 and SF6, which can remain in the atmosphere for over 50,000 years before degrading. These two gases are used in the plasma reactors required to fabricate computer chips. To avoid damaging the pumping system, the fluorinated gases were “drowned” in a nitrogen stream requiring plasma effluents carried in rapid nitrogen flows.
The process, which takes place at atmospheric pressure with the addition of a few per cent of oxygen (oxidizing the fragmented molecules, preventing them from reassociating and giving them an acid-like nature facilitating their scrubbing on an alkaline bed), is ecologically clean and electrically very efficient when compared to burners previously used. These systems are employed industrially in abating fab- reactor greenhouse gases as well as purifying noble gases (6).
3. Sterilising medical equipment
Sterilisation implies that at the most, only a single microorganism is left among initially one million. A surfatron, a lower-frequency microwave field applicator (915 MHz), is used rather than the surfaguide where 200 W is absorbed in the 50-litre sterilization chamber (8).
An optimised mixture of N2 and O2 is used to generate a plasma column of NO excited molecules that emit an intense afterglow of ultraviolet light: the afterglow designates the region far enough from the surfatron where no electric field from the surface wave is present and, sufficiently farther along it, to reach the late-afterglow domain where there are no more electrons. Ultraviolet light with wavelengths of between 180 and 350 nanometres induces lesions in the DNA of microorganisms, ultimately when these are numerous enough to lead to their inactivation.
The advantage of the present SW sterilisation technique over all other plasma sterilisation systems is that it relies on the far flowing- afterglow (Fig. 3). This is an original and unique feature of the late-afterglow method since with all other plasma sterilization techniques electrons charge up on microorganisms, which are then released by an electrostatic force acting on the substrate so rapidly that they do not become inactivated, eventually contaminating the sterilization chamber and flowing out into the “outside world” (9).
Compared to chemical sterilization processes (e.g. ethylene oxide), no post-process vent time is required with the afterglow technique since no toxic components have entered polymeric materials, ensuring absolutely safe sterilization, e.g. for contact lenses.
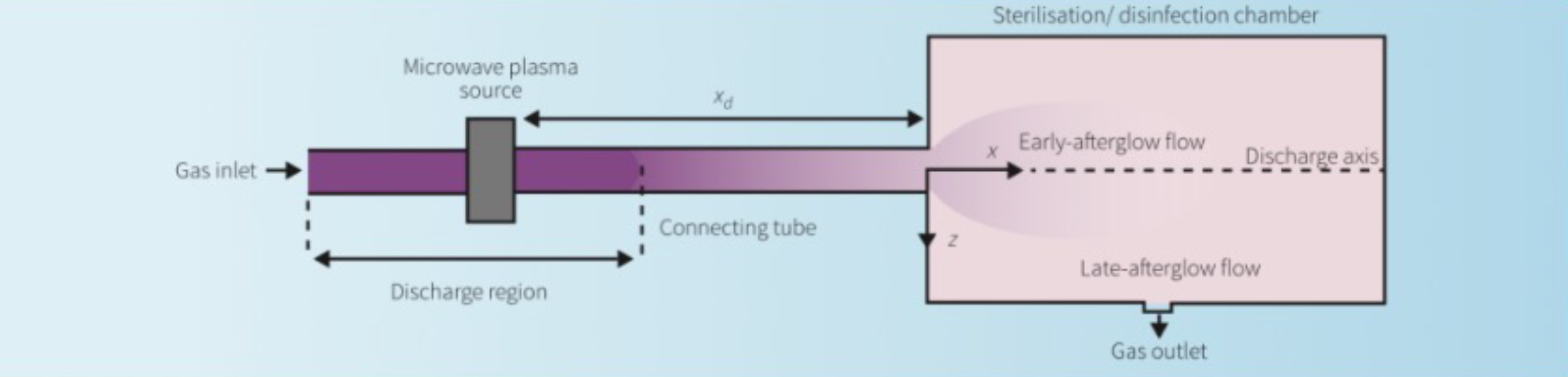
The development of new applications based on plasma produced by microwave fields is now facilitated by the recent publication of a fluid (hydrodynamic) model (10) allowing us to better understand the particular kinetics of the electrons in these plasmas and to benefit from them: only the electrons are accelerated by the microwave electric field. These “electronic” plasmas are complementary to RF plasmas where the important role of ions is distinctive, by the presence of ionic sheaths offering to benefit from the directionality of the ionic bombardment without which it would not be possible to manufacture microelectronic chips.
On the other hand, the treatment of gases by microwave fields seems to offer a huge field of applications that were previously unimaginable. For example, the decomposition of CO2 gas, which is prevalent on Mars, could make it possible to obtain the oxygen necessary for the establishment of “terrestrial” life bases on this distant planet. (ref.)
References
- I. Zhelyazkov, E. Benova and V. Atanassov (1986) Axial structure of a plasma column produced by a large-amplitude electromagnetic surface
wave, Journal of Applied Physics 59, 1466-1472. - S. Schelz, C. Campillo, M. Moisan (1998), Characterization of diamond films deposited with a 915-MHz scaled-up surface-wave-sustained
plasma, Diamond Relat. Mater. 7 1675-1683. - Moisan M., Nowakowska H. (2018) Contribution of surface-wave (SW) sustained plasma columns to the modeling of RF and microwave discharges with new insight into some of their features. A survey of other types of SW discharges, Plasma sources science and technology, 27, 073001, 43 pages.
- Benova et al. (2022) Characteristics of 2.45 GHz Surface-Wave-Sustained Argon Discharge for Bio-Medical Applications. Appl. Sci. 12, 969-984.
- Bertrand L., Monchalin J.-P., Pitre R., Meyer M.L., Gagné J.M., Moisan M. (1979) Design of a compact CW chemical HF/DF laser using a
microwave discharge. Rev. Sci. Instrum., 50 708-713. - Kabouzi Y., Moisan M., Rostaing J.C., Trassy C., Guérin D., Kéroack D., Zakrzewski Z. (2003) Abatement of perfluorinated compounds using
microwave plasmas at atmospheric pressure. J. Appl. Phys., 93 9483-9496. - Moisan M. Scientia 2023…
- Moisan M., Boudam K., Carignan D., Kéroack D., Levif P., Barbeau J., Séguin J., Kutasi K., Elmoualij B., Thellin O., Zorzi W. (2013) Sterilization/disinfection of medical devices using plasma: the reduced-pressure flowing-afterglow of the N2-O2 discharge as the inactivating medium, European Physical Journal: applied physics, 63 10001, 46 pages.
- Moisan M., Levif P., Séguin J., Barbeau J. (2014) Sterilization/disinfection using reduced-pressure plasmas: some differences between direct exposure of bacterial spores to a discharge and their exposure to a flowing afterglow, Journal of Physics D: applied physics, 47, 285404, 14 pages.
- Moisan M., Ganachev I.P., Nowakowska H. (2022). Concept of power absorbed and lost per electron in surface-wave plasma columns and its contribution to the advanced understanding and modeling of microwave discharges, Physical Review E, 106, 045202.

This work is licensed under Creative Commons Attribution-NonCommercial-NoDerivatives 4.0 International.