In this piece, Dr Helen Rowe summarizes how arrays or strings of multi-copy satellite DNAs can barcode chromosomes to regulate cell fate, by acting as molecular switches
While we have gained extensive knowledge about what our genes do and how mutations occurring in them can lead to diseases such as cancer, less is known about genomic ‘dark matter’.
Dark matter refers to DNA of unknown function and makes up a huge 98% of our genome. Intense research in this area has revealed that dark matter contributes to gene-regulatory networks that serve to control where and when sets of genes are switched ON or OFF. Specialised cell types work by each expressing a unique set of genes: for example, a cell that functions in the adaptive immune system, such as an activated T cell, will switch on a different subset of genes than a neuron in the brain. Likewise, development is a highly regulated process, whereby different sets of genes are progressively switched on in different tissues. Thus, the dark matter within our genome contributes to the control of gene regulatory networks and biological systems.
Satellite DNAs and how they can switch ON host genes?
Dark matter is comprised of repetitive DNA, which describes DNA sequences that are reiterated in multiple copies throughout the genome. Some repetitive DNA is derived from virus-like transposable elements that were once mobile and replicated in the genome, leaving a fossil record of interspersed repeats. Satellite repeats, in contrast, are short units of repetitive DNA that have expanded in tandem leading to the formation of satellite DNA arrays.
Centromeres that provide structure to chromosomes are made of densely packed satellite DNAs. However, other satellite DNA arrays (including minisatellites and microsatellites) are scattered across our chromosomes and have been poorly studied. This is partly because their repeated units are short, fairly common and appear insignificant, when positioned next to well-annotated and studied cellular genes. When you look at broader chromosomal regions, it is apparent that satellite DNAs can form discrete arrays (or barcodes) that are megabases in size and could act as key regulatory hubs. Examination of one of these repeat units with reference to the epigenome, combined with functional assays has shown that each unit harbours an enhancer element. The entire array, therefore, acts as a multicopy enhancer, which we refer to as a ‘super enhancer’ here. Moreover, embedded within each repeat unit are ‘repressor’ sites. This means that the array can act as a molecular switch that could be turned on and off.
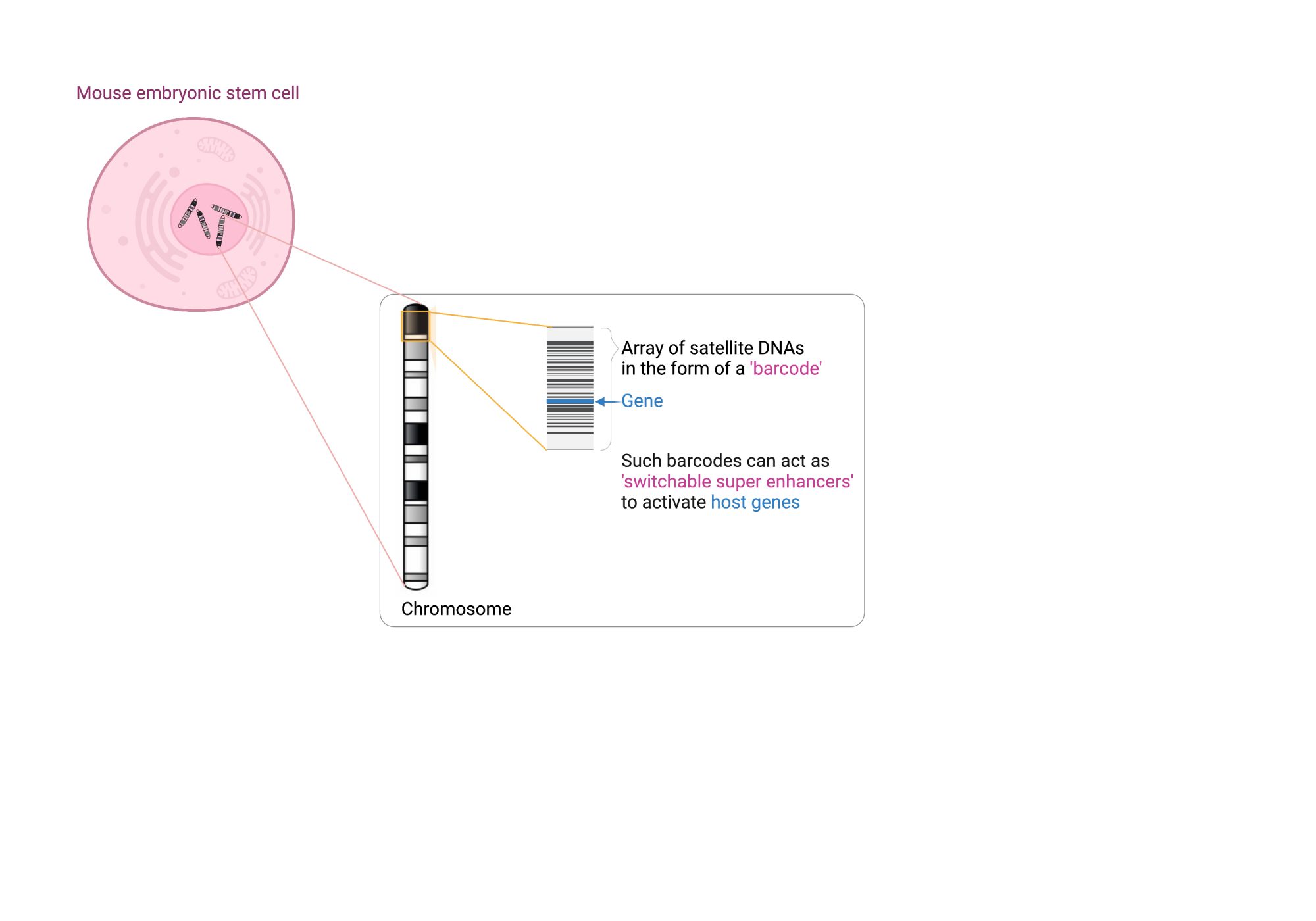
What are KRAB-ZFPs (KZFPs) and how do they control molecular switches?
Transcription factors home in to target genomic sites in a sequence-specific way. We and other labs have discovered some of these proteins, known as KRAB-zinc finger proteins (or KZFPs) to specifically target viral-like DNA, including satellite repeats. KZFPs switch off repeats by recruiting chromatin-associated proteins, such as TRIM28 and SETDB1 to create local heterochromatin (silent chromatin). Viruses and their hosts compete in an evolutionary arms race, with the host continually needing to find new defence mechanisms to block invading viruses. Because of this, KZFP proteins likely first evolved to block genome invasions and to combat the unstable expansions of satellite repeats across chromosomes. Over time, this mechanism of dynamic heterochromatin formation has been adopted by the host as a molecular switch to regulate genes.
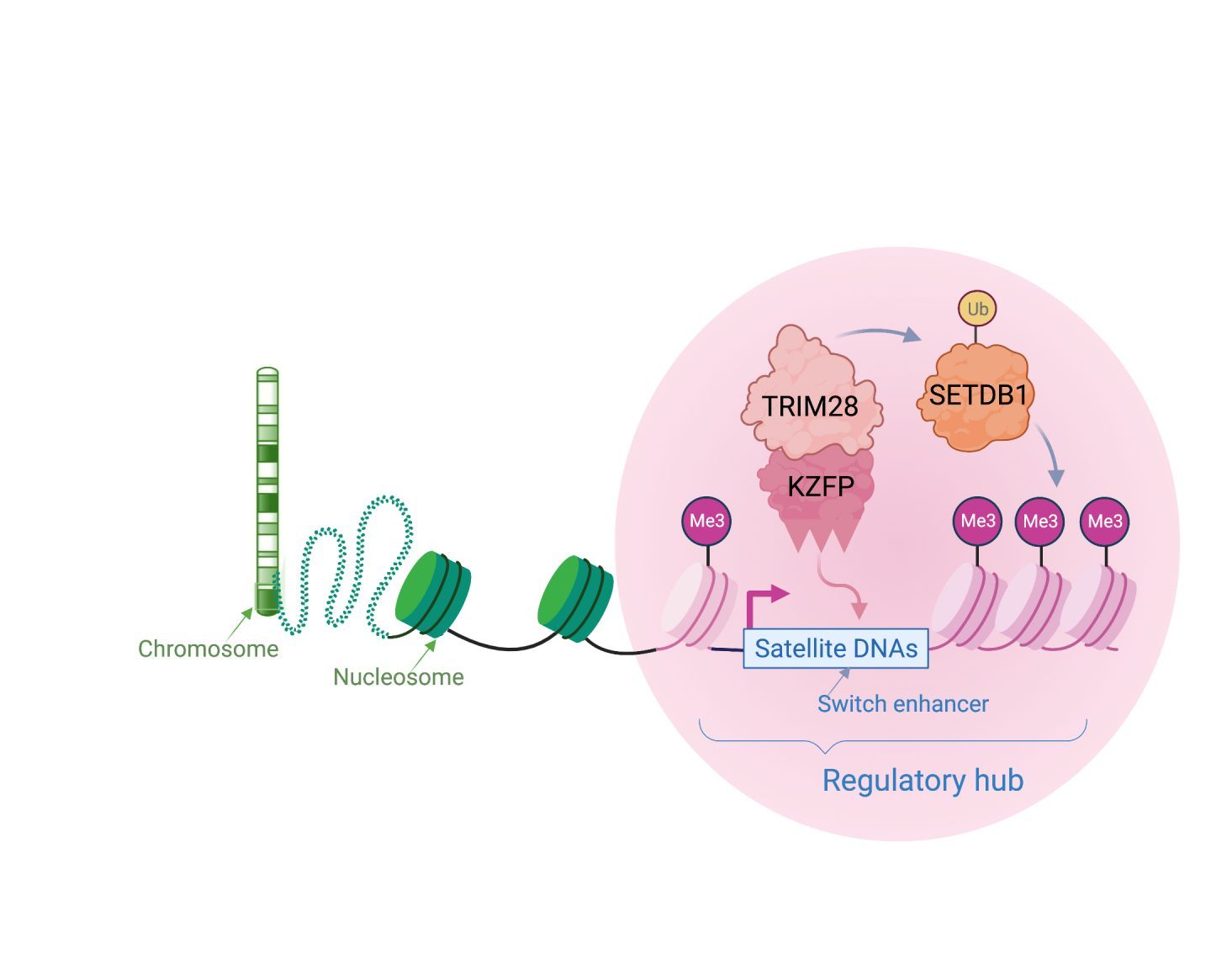
Molecular switches are cis-regulatory hubs that recruit dynamic protein complexes to remodel chromatin state. This leads, for example, to a shift from gene repression to activation.
We have uncovered a molecular switch that controls a mouse gene that is a master regulator of cell fate (Zscan4). When it becomes switched on, it can activate a whole set of genes that are needed in early mouse development. This satellite DNA array can also regulate heterochromatin more broadly through its cognate KZFP (known as ZFP819). This suggests that satellite DNA arrays can remodel chromatin to direct cell fate changes.
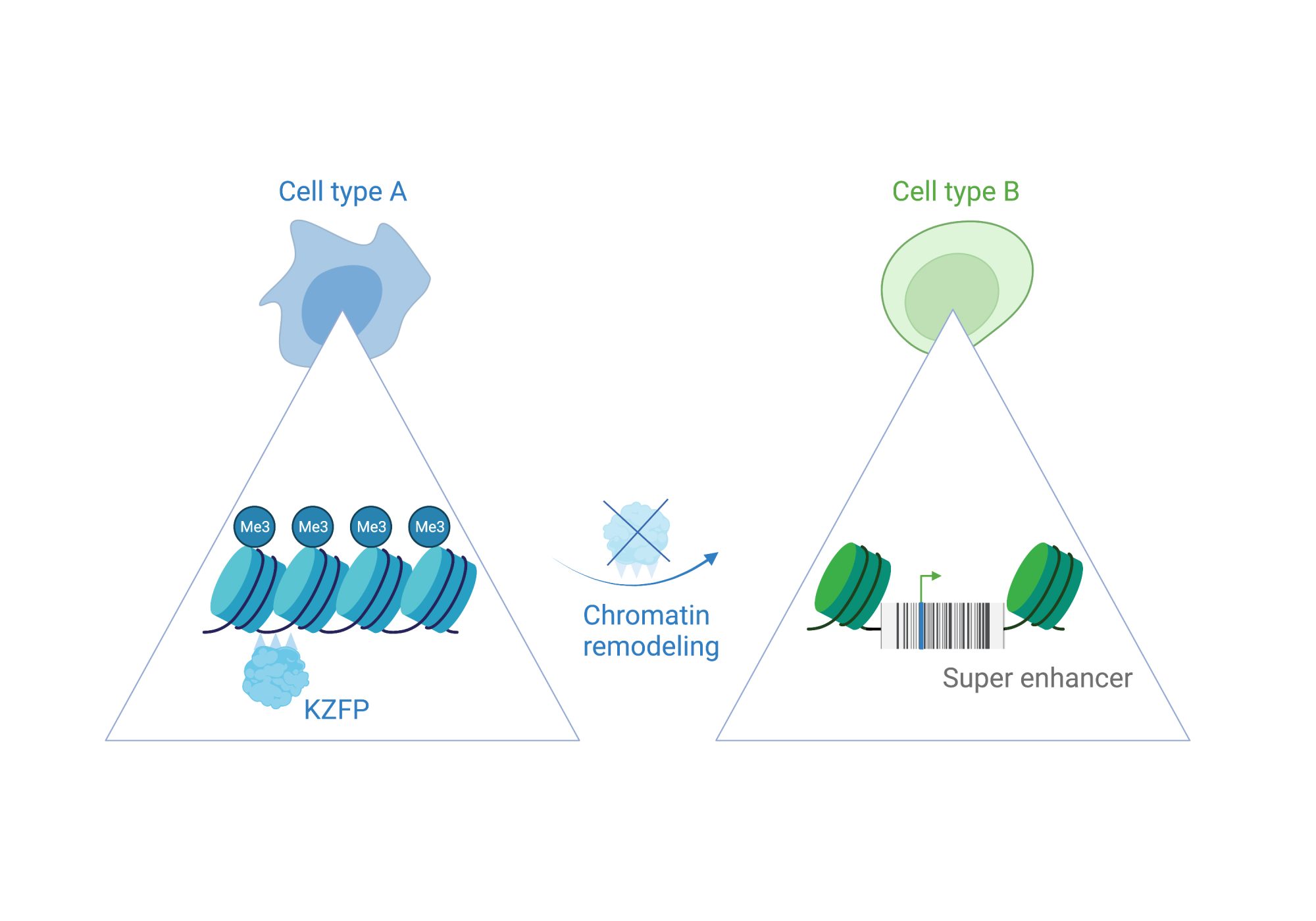
How do satellite DNA arrays relate to human disease?
Although we have identified a satellite repeat barcode that acts as a molecular switch in the mouse genome, by analogy we hypothesize that human satellite repeats will act in a similar fashion. Unwarranted activation and instability of human satellite DNAs is a feature of many cancers and is associated with global chromatin remodelling events. The heterochromatin in our cells is important to maintain structure and cell identity because it acts as a roadblock to transcription and aberrant gene activation.
The molecular switch that we have uncovered is at the heart of the maintenance of heterochromatin, the loss or depletion of which can lead to cell fate changes. A hallmark of cancer cells is their ability to adopt a more de-differentiated state with increased genome plasticity and proliferation. In the future, we hope to understand how chromatin remodelling is controlled in human cells and how this could be linked to cancer progression.
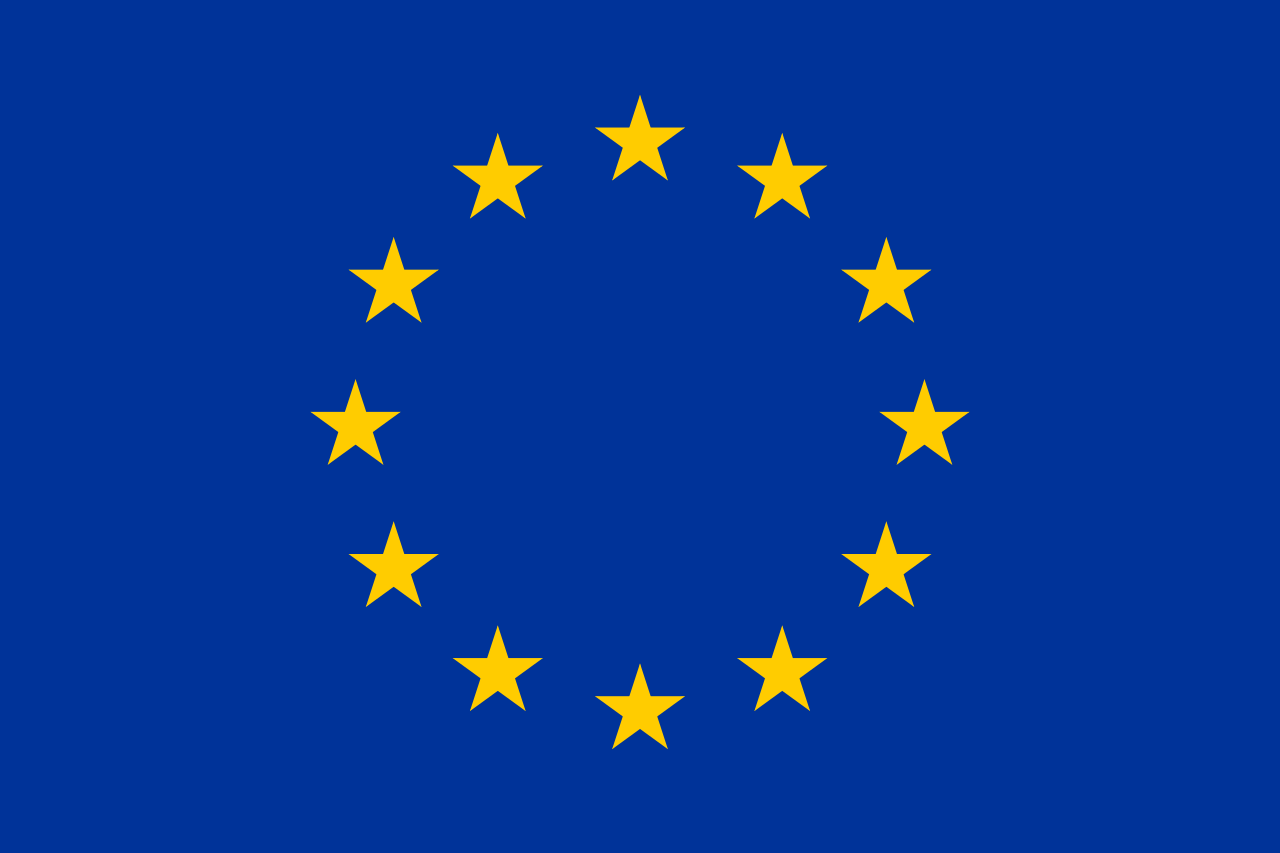

This work is licensed under Creative Commons Attribution-NonCommercial-NoDerivatives 4.0 International.