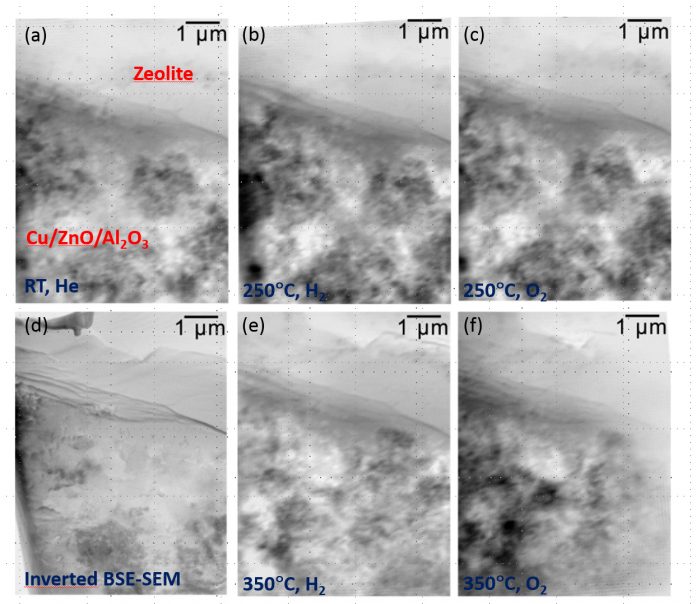
Christian Danvad Damsgaard at DTU Cen provides an insight into in situ investigations of operating catalysts by combining x-ray based characterisation with electron microscopy
Fundamental insight into structure-functionality relationships is key to understand the function of catalysts and improve catalytic properties. As catalysts may change their structure with respect to the environment, it is essential to investigate the catalysts under reaction conditions. Furthermore, structural and compositional information has to be acquired on different length scales (1) and such in situ studies require dedicated complementary techniques.
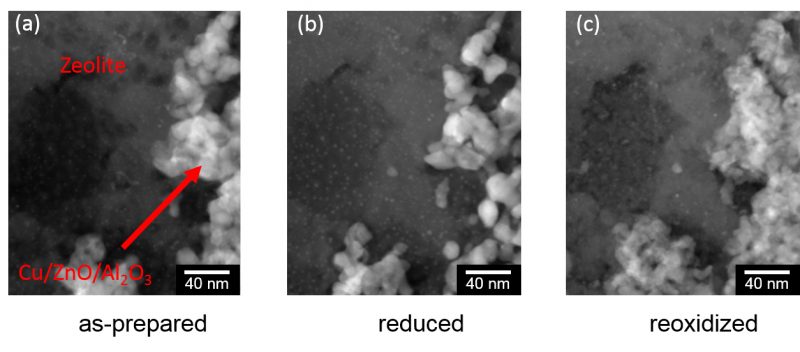
In situ x-ray diffraction (XRD) and x-ray absorption spectroscopy (XAS) is eminent to follow the catalyst’s average structural and electronic changes during synthesis and reaction conditions (2). In order to follow the dynamics (stability, topography, etc.) of the surface and interfaces, imaging is often the solution (3). Nanoscale imaging and spectroscopy of catalysts in a gaseous environment are usually performed in an environmental transmission electron microscope (ETEM) (Figure 1). TEM gives insight in the structural changes at the atomic scale during the reaction, however, it is restricted to relatively low pressure (<~1 kPa) and a thin sample (<~100 nm) (4). Recent developments provide special sample holders with nano-reactor in which the sample can be exposed to conditions around 100kPa.
Spatially resolved information on the mesoscale (50 nm – 1 µm) can be obtained by x-ray microscopy, which enables in situ studies at both ambient and elevated pressure (6). Furthermore, due to the much higher penetration depth of x-rays compared to electrons, x-ray based characterisation techniques are more suited for realistic sample environment and geometry. By use of a specially designed in situ cell, catalytic systems can be studied with hard x-ray microscopy (x-ray ptychography) (7) during the reaction (Figure 2). The in situ cell is based on a MEMS-based TEM heater chip, which enables ex situ electron microscopy imaging before and after reaction (6).
Figure 1 and 2 illustrate the complementary nature of in situ hard x-ray ptychography and electron microscopy applied on a Cu/ZnO@zeolite core-shell catalyst for direct production of dimethyl ether. The combination of ETEM results, obtained at a low pressure of a thin ~100nm slice, with ptycography results, obtained at a high pressure of a thicker 300-400 nm slice, enables bridging of both material gap (thin vs. thicker) as well as pressure gap. The study reveals a stable core-shell interface at 250°C, although reduction of the Cu containing core material led to a shrinkage of the particles on the nanometer scale. At further heating to 350°C, changes on the μm scale were observed. The catalytic performance of the catalysts was not characterised in the above study as the reactor volume to sample volume is too large in the current in situ cell and the ETEM.
Results
The results underline the need for complementary techniques and highlight the potential of these for application in catalysis. Future developments should be focused on closing the material gap, as well as the pressure gap and make it possible to study catalysts with complimentary methods under directly comparable conditions such as pressure, temperature and gas flow. Furthermore, actions should be taken towards measuring the activity of the catalyst, such as for example, mass spectrometry to clearly and without any doubt be able to state that the measurements were performed on a working catalyst.
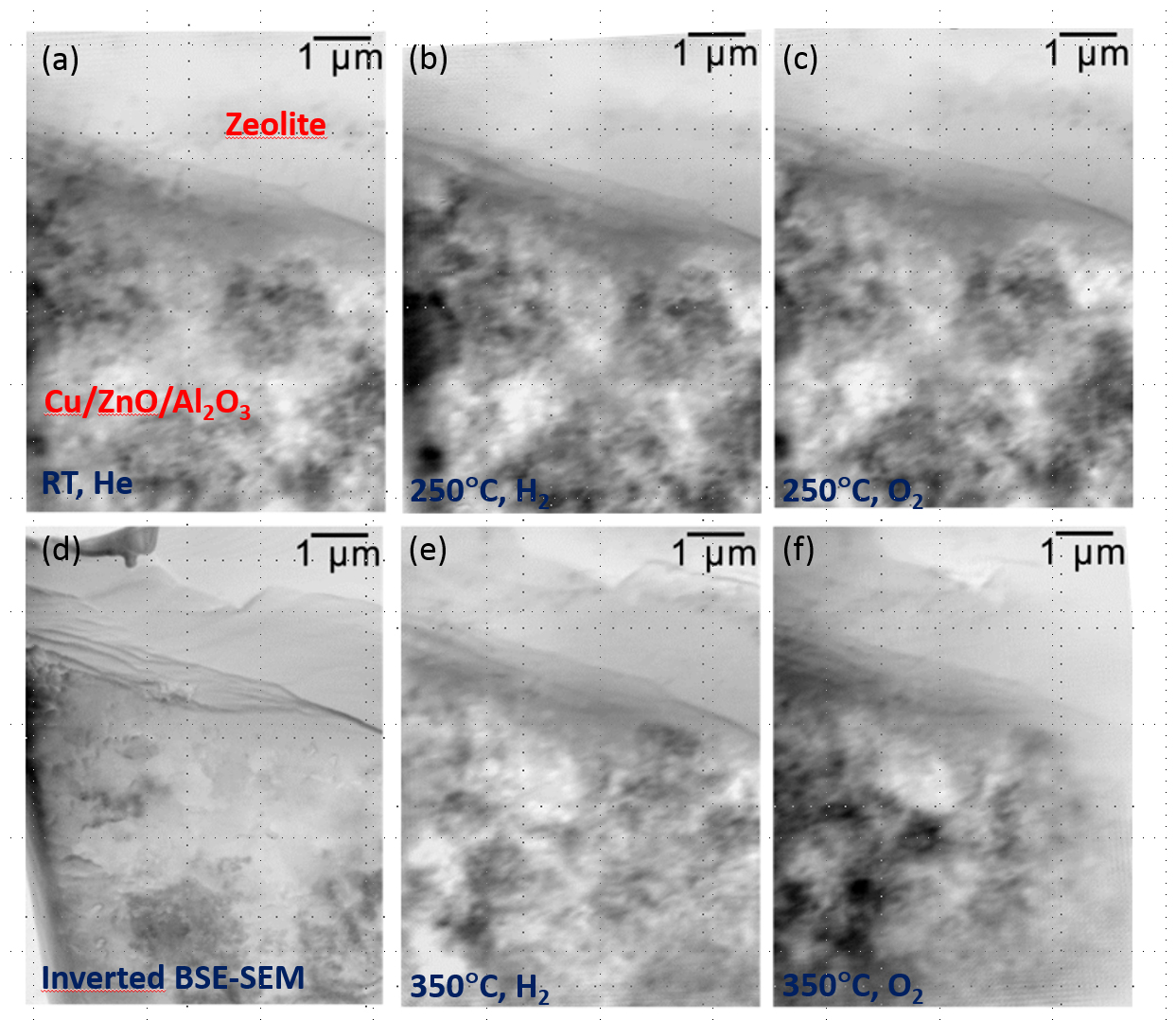
Figure 2: In situ ptychograms (phase contrast) at 105Pa of a 300-400nm slice of a Cu/ZnO@zeolite core-shell catalyst at room temperature in He (a), 250°C in H2 (b), 250°C in O2 (c), 350°C in H2 (e), and 350°C in O2 (f), respectively. (d) inverted backscattered electron (BSE)-scanning electron microscopy (SEM) image showing material with a high atomic number as darker areas. The BSE-SEM images were recorded ex situ before the in situ x-ray ptychography treatment of the sample7
References:
1 J. D. Grunwaldt, J. B. Wagner, and R. E. Dunin-Borkowski, “Imaging Catalysts at Work: A Hierarchical Approach from the Macro- to the Meso- and Nano-scale,” ChemCatChem, vol. 5, no. 1, pp. 62–80, 2013.
2 B. S. Clausen, “Combined (Q)EXAFS/XRD: Technique and Applications,” Catal. Today, vol. 39, no. 4, pp. 293–300, 1998.
3 Q. Jeangros, T. W. Hansen, J. B. Wagner, R. E. Dunin-Borkowski, C. Hébert, J. Van Herle, and A. Hessler-Wyser, “Measurements of local chemistry and structure in Ni(O)-YSZ composites during reduction using energy-filtered environmental TEM.,” Chemical communications (Cambridge, England), 2014. [Online]. Available: http://www.ncbi.nlm.nih.gov/pubmed/24400316. [Accessed: 08-Jul-2015].
4 T. W. Hansen, J. B. Wagner, P. L. Hansen, S. Dahl, H. Topsøe, and C. J. H. Jacobsen, “Atomic-Resolution in Situ Transmission Electron Microscopy of a Promoter of a Heterogeneous Catalyst,” Science, vol. 294, no. 5546, pp. 1508–1510, 2001.
5 J. F. Creemer, S. Helveg, G. H. Hoveling, S. Ullmann, a. M. Molenbroek, P. M. Sarro, and H. W. Zandbergen, “Atomic-scale electron microscopy at ambient pressure,” Ultramicroscopy, vol. 108, no. 9, pp. 993–998, 2008.
6 S. Baier, C. D. Damsgaard, M. Scholz, F. Benzi, A. Rochet, R. Hoppe, T. Scherer, J. Shi, A. Wittstock, M. Burghammer, J. B. Wagner, C. G. Schroer, and J.-D. Grunwaldt, “In Situ Ptychography of Heterogeneous Catalysts using Hard x-Rays: High Resolution Imaging at Ambient Pressure and Elevated Temperature,” Microsc. Microanal., vol. 22, pp. 178–188, 2016.
7 S. Baier, C. D. Damsgaard, M. Klumpp, J. Reinhardt, T. Sheppard, Z. Balogh, T. Kasama, F. Benzi, J. B. Wagner, W. Schwieger, C. G. Schroer, and J.-D. Grunwaldt, “Stability of a bifunctional Cu-based core@zeolite shell catalyst for dimethyl ether synthesis under redox conditions studied by environmental transmission electron microscopy and x-ray ptychography,” Microsc. Microanal., vol. 23, no. 3, pp. 501–512, 2017.
Please note: this is a commercial profile
Christian Danvad Damsgaard
Associate Professor
Center for Electron Nanoscopy
DTU Cen, Technical University of Denmark
Tel: +45 4525 6487