Regular exercise is a critical step in maintaining healthy physiology and ensuring healthy aging. However, there are many diseases and conditions that make exercise inaccessible or reduce its efficacy. The Wessells lab studies exercise and the pathways it works through to identify key molecules required for a proper exercise response
They are now focusing on applying this knowledge to better understand how to bring the benefits of exercise to those who may be unable to exercise and to those who may not experience the normal physiological improvements that come from regular exercise.
The Wessells lab studies exercise using the fruit fly Drosophila as a genetic model due to its short lifespan, large sample sizes, and low cost of maintenance. Moreover, about 60% of fly genes have known human homologs, making genetic discoveries highly likely to be relevant to humans.
The interactions between exercising muscle and the brain are also similar across species, making discoveries in flies likely to be physiologically relevant to humans as well. Currently, the lab is actively investigating the relationship between exercise and circadian rhythms and between exercise and memory.
Exercise and circadian rhythms
Most animals, including humans, experience a daily cycle of gene expression and metabolic activity known as circadian rhythm. Many people experience frequent disruptions to their circadian rhythm, including shift workers, jet lagged travelers, patients on certain medications, and those with disrupted sleep patterns. Chronic disruption of circadian rhythm can lead to declines in cardiovascular, mental or metabolic health.
Experiments in humans and lab animals have shown that exercise can help to maintain circadian rhythms. Unfortunately, exercise is often less accessible to such patients, because of their difficult schedules. Compounding this problem, disrupted circadian rhythms can reduce physical endurance, making it more difficult to complete rigorous exercise programs. The Wessells group has set a goal to utilize molecules activated by exercise to induce benefits of exercise in flies experiencing rhythm disruptions, despite their reduced endurance. In doing so, they hope to identify targets for pharmaceutical interventions to bring these benefits to humans.
Rescuing exercise ability in flies with disrupted circadian rhythm
To do this, they used a fly mutant in a gene called clock as a model of circadian disruption. The Clock protein is an important molecule for regulating the circadian rhythmic “clock” in the brain of both flies and humans. Thus, clock mutants have permanently disrupted circadian rhythms and abnormal sleep patterns.
They found that the clock mutant had a reduced exercise performance in several assessments, including endurance, climbing speed, and flight performance. They also found that, much like mice or humans with disrupted circadian rhythms, the mutant flies were unable to respond to an exercise training program by improving their speed and endurance.
Molecularly, they found that the mutant had reduced expression of several genes that are known to be critical for improvements during exercise training, including sestrin, a conserved regulator of protein turnover and glucose metabolism, spargel, a conserved regulator of mitochondrial biogenesis and fatty acid metabolism known as PGC-1α in humans, and Iditarod, a conserved regulator of metabolism known as FNDC5/Irisin in humans, which pointed to mechanistic reasons that these animals could not improve with training.
Prior work from the lab had established that the exercising brain in flies releases a norepinephrine-like molecule called octopamine, and octopamine travels in the circulation back to the muscle to change gene expression there. They hypothesized that stimulating clock mutants with octopamine might restore their exercise ability by activating the expression of genes like sestrin and spargel in muscle. Since octopamine works in the circulation, they could give octopamine by simply feeding it to the flies in a carefully controlled dosage.
Octopamine feeding indeed rescued the ability of clock mutants to perform endurance exercise, with speed and endurance both increased back to normal levels, an exciting result. However, it was important to find out what the downstream effectors of octopamine were in order to translate these results to humans, because upregulation of the human equivalent of norepinephrine would lead to chronically increased blood pressure, making it an unattractive therapeutic option.
It turned out that octopamine also increased the expression of the exercise response genes sestrin, spargel and Iditarod, consistent with the idea that upregulating these important genes for exercise could hold the key to restoring exercise capacity in humans with disrupted circadian rhythms. Importantly, flies fed octopamine regained these abilities without curing their circadian rhythm disruption, which implies that eventual treatments targeting these molecules in humans could restore exercise capacity even if sleep and metabolism remain disrupted by shift work and/or frequent international travel.
Next steps will be to use genetic tools available in the fly model system to manipulate each of the exercise response genes separately to ascertain whether any of these by itself could be sufficient to restore exercise ability. This is important because narrowing down to a single molecule will facilitate identification of drugs that safely target these molecules as possible therapeutics.
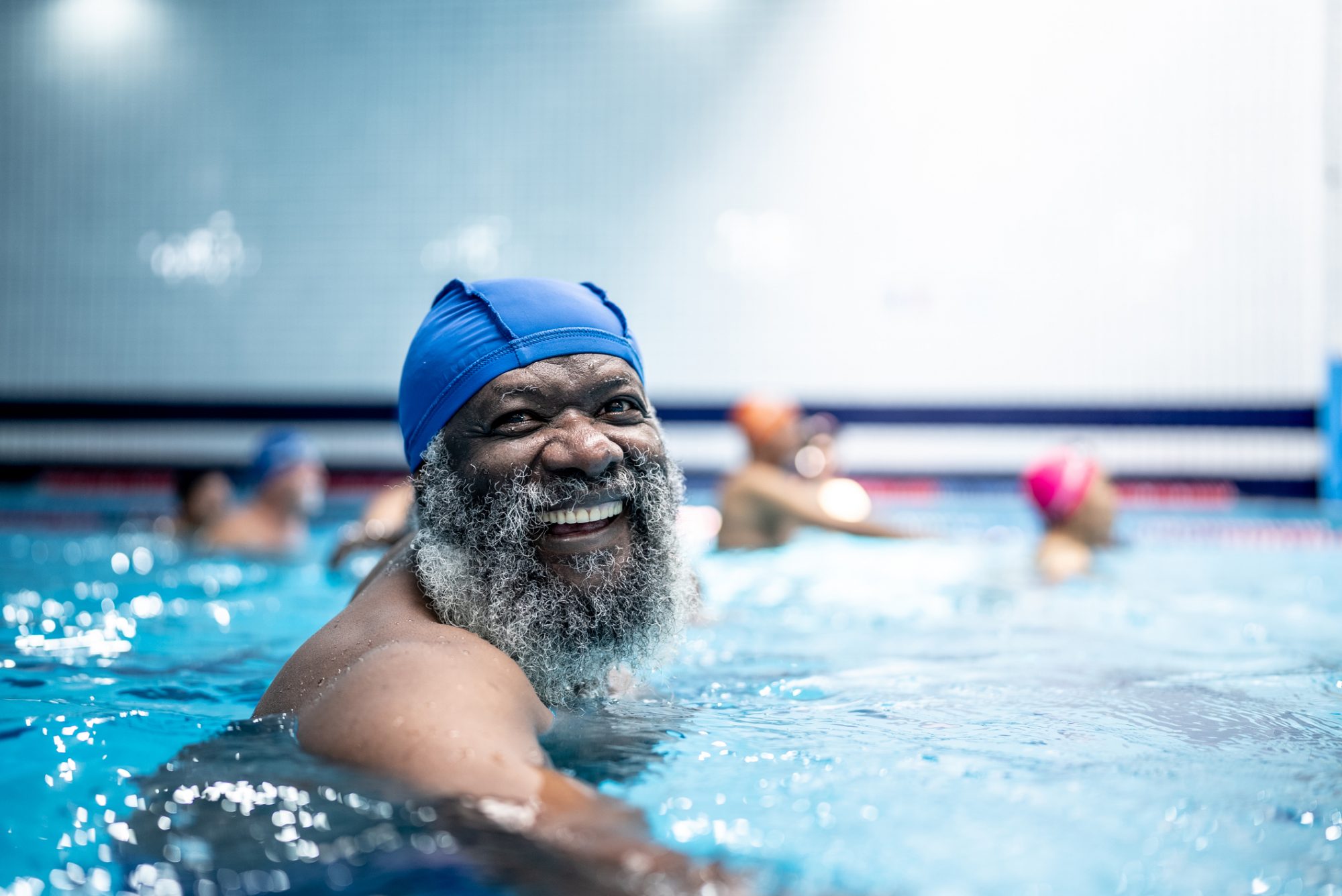
Benefits of regular exercise in protecting against cognitive decline in aging
Gradual decline in cognitive speed and memory as a consequence of normal aging, has been observed in most model organisms, and in the human population. Conversely, regular exercise has been observed in epidemiological studies to protect human cognitive abilities and to slow age-related declines. Similar results have been found in laboratory mice, suggesting that the protective effects of exercise act through molecular mechanisms that are conserved across species.
However, the specific molecules and pathways that mediate the protective effect of exercise against cognitive decline have yet to be thoroughly worked out. Once these molecular pathways have been identified, they will serve as rational targets for therapeutics to protect memory across the aging population.
The fly system is a perfect model to investigate this important topic, since it has well-established methods for measuring memory across the lifespan, and also has the methodology developed by the Wessells lab to do controlled exercise training across ages. In addition, using flies for this purpose allows access to the many genetic tools that have been developed for use in flies over the decades.
Initial experiments show that regular exercise throughout life does indeed significantly slow the normal memory decline during aging in wild-type flies, although it does not completely eliminate the effect of age. The next steps are for the lab to utilize the molecules they have identified that can mimic the effects of age, to see if those molecules by themselves could preserve memory, even when the animal is not performing regular exercise.
Does it make sense that octopamine, for example, could have a protective effect on memory? There are several reasons to believe that it might. The human equivalent of octopamine, which is norepinephrine, has been associated with the stabilization of memories in mice and humans. It is also worth noting that octopamine, like norepinephrine, stimulates a signaling pathway involving cyclic AMP (cAMP), and this signaling pathway has been associated with memory formation and retention in both flies and mice.
The Wessells lab has extensively studied the signaling effects of octopamine after it is released from neurons and is received in muscle. They are currently working to extend these studies to the signaling effect of octopamine to other neurons in the brain, where they believe octopamine is likely acting to stimulate neural circuits involved in memory. Interestingly, prior experiments have already established that octopamine receptors are required in the brain for basic effects of exercise on speed and endurance, so it is reasonable to expect that signals from octopamine are required for changes in brain function during exercise as well.
Molecules induced downstream of octopamine in exercising muscle, such as sestrin and Iditarod, are also important in the brain. In fact, others have shown that sestrin overexpression in neurons can slow disease progression in a fly model of Parkinson’s Disease, and the mouse homolog of Iditarod has been associated with exercise-induced cognitive protection, supporting the idea that the exercise-response genes important in the brain are similar to the genes that are important in muscle.
Exercise-induced protection against Alzheimer’s disease
While normal aging frequently (though not always) results in cognitive declines, these declines are much more extreme in patients with Alzheimer’s disease (AD). AD patients are often unable to lead a normal life due to substantial memory loss, confusion, and spatial difficulties that are associated with the presence of amyloid plaques. Amyloid plaques are aggregates of misshapen proteins that form between nerve cells and can act as roadblocks for memory.
Cell death also occurs in specific areas of the brain, including the hippocampus, that are critical for areas of cognition like memory and spatial navigation. Therapeutic interventions that can preserve cognitive function in AD are therefore of substantial importance, particularly because the incidence of Alzheimer’s Disease diagnoses is expected to grow as a function of the increase in elderly populations.
One promising intervention that protects cognition in AD patients is regular endurance exercise. Endurance exercise can benefit brain health by triggering the release of growth factors such as Brain Derived Neurotrophic Factor (BDNF) – chemicals that affect the growth of new cells in the brain and can protect existing cells from cell death. Furthermore, regular exercise has been associated with reduced epidemiological risk of AD and improved cognitive function in AD patients.
However, there are still gaps in our understanding of the mechanisms by which endurance exercise improves cognitive function in AD. Also, many AD patients are unable to exercise because of their advanced age or mobility decline. Understanding the mechanisms of exercise in protecting against cognitive decline of AD is key to maximizing the potential of exercise response molecules as therapeutic treatments to preserve memory.
Fly models for AD have been established and successfully utilized over the years to generate important mechanistic advances. These models show disease progression moving from memory loss to neurodegeneration, neuronal cell death, and early mortality, similar to mouse models and human patients in many important ways.
The Wessells lab is now beginning to utilize their fly exercise system to first establish that exercise shows the same protective effects in the fly that it does in mice and humans. The next step will be to use the exercise response pathways discussed above to find out if these can not only protect memory against normal aging, but also protect against accelerated memory loss and cell death in neurodegenerative diseases such as AD.
How likely is it that the octopamine/sestrin/ Iditarod axis will be protective against this deadly disease? Again, there are solid reasons to believe it might be. First, the activity of the mouse homolog of Iditarod has been shown to stimulate the protective effects of BDNF in the brain. Second, expression of sestrin in neurons has been shown to slow disease progression in models of other diseases that are characterized by protein aggregates in neurons, including Spinal Cerebellar Ataxia 2 and Kennedy’s Disease.
Based on this rationale, the lab is currently examining whether neuronal upregulation of octopamine release, sestrin expression or Iditarod expression can provide substantial protection against memory loss and neuronal cell death in fly models of AD.
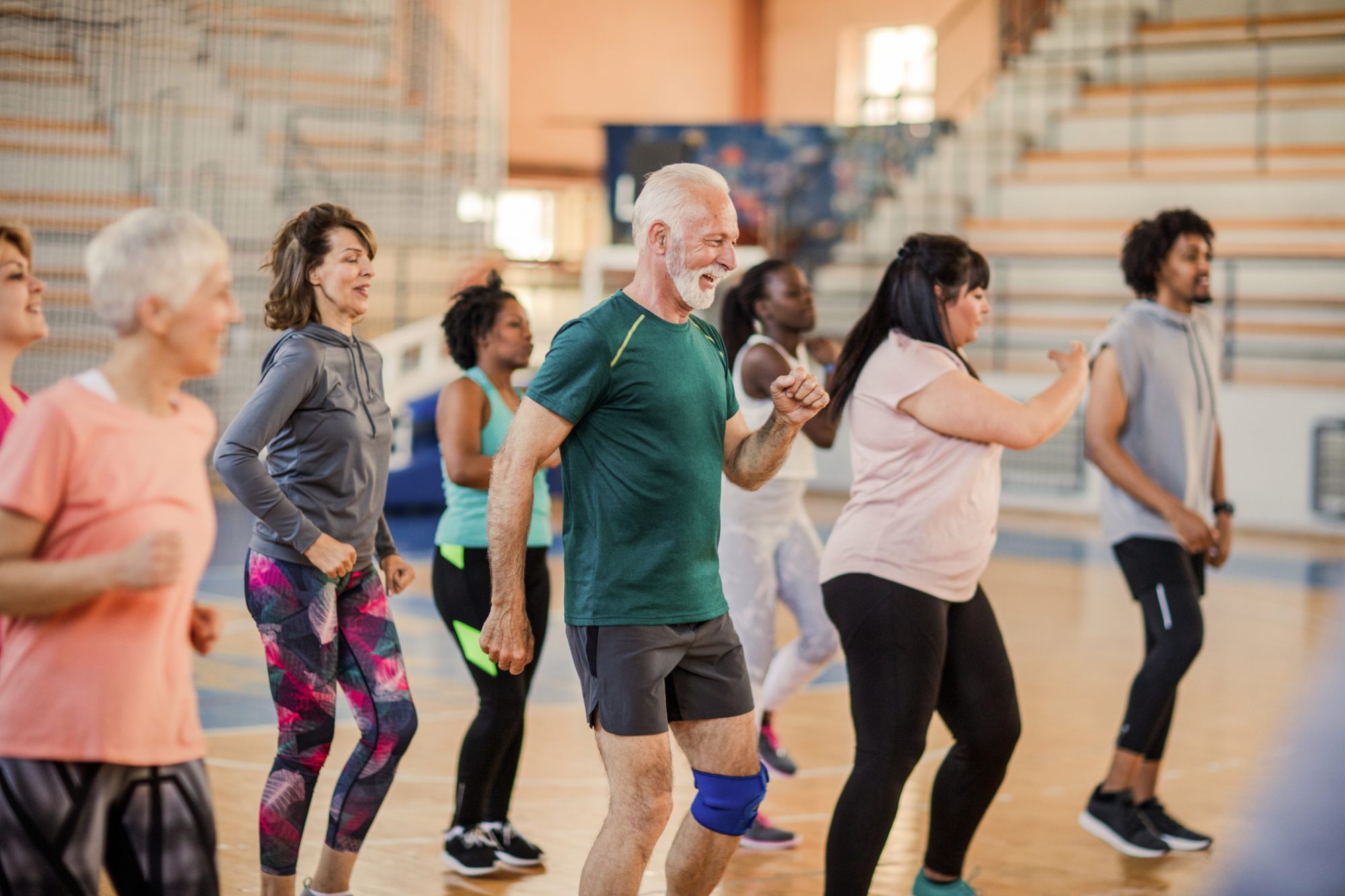
Use of virtual reality to Stimulate Neuroprotection
Based on findings from the Wessells lab and others demonstrating that the physiological response to regular exercise is regulated by the brain, the idea has arisen that it may be possible to stimulate the brain with sensory input that “tricks” the brain into thinking a patient is exercising.
One way to do this is by using virtual reality (VR). Several labs have shown that VR gives patients the sights and sounds of exercise can increase heart rate and norepinephrine (human version of octopamine) release. The Wessells lab is currently studying whether using VR can stimulate improvements in glucose sensitivity even when the study volunteer is not doing any actual exercise.
A major obstacle to using exercise as a treatment to slow progression of AD is the fact that many patients with AD have reduced exercise capacity as a result of the disease, and also the advanced age of many such patients. In the future, it may be possible to use VR to stimulate the physiological response to exercise even in patients that are physically unable to perform intense exercise. Such an application would be highly transformative in the lives of patients.
In summary, exercise response pathways identified through the study of exercising fruit flies are steadily opening new doors to examine potential treatments for many conditions in humans. The years to come are expected to continue bringing this work closer to its application to humans badly in need of effective treatments.