Livestock are an important source of food for the planet’s eight billion humans; societies across the globe must work together to meet the ever-increasing demand for food through reproductive technologies, if we hope to attain global food security
Animal-source foods are a vital component of a healthy well- balanced human diet, providing a much-needed source of protein (including specific amino acids not commonly found in plant-based diets), energy, minerals, and vitamins.1
The world’s population is growing faster than ever before, and it is imperative that societies across the globe work together to meet the ever-increasing demand for food if we hope to attain global food security. The United Nations Food and Agriculture Organization states that global food security exists when “all people at all times have physical, social, and economic access to sufficient, safe, nutritious food that meets their dietary needs and food preferences for an active and healthy life”.2
The changes needed to achieve global food security include (but are not limited to) reducing pre-harvest and post-harvest loss of food, improving the distribution of food, decreasing wasted food, and increasing food production in an environmentally sustainable manner. Concurrently, reductions in greenhouse gas emissions from the transportation, energy, manufacturing, and agriculture sectors are needed to better cope with climate change.
Livestock contribute more to communities than just food
In many developing nations, livestock not only serve as a source of food but also provide draft power, transportation, companionship, fiber for clothing, support of cultural and religious practices, and storage of capital (which contributes to the resilience of smallholder farmers). Livestock contribute to soil fertility enhancement, provide ecological benefits (pest & weed control), and serve both as an important source of income – especially for women – as well as a financial asset that is often overlooked in rural economic development. Ruminant livestock (e.g., sheep, goats, and cattle) convert non-edible low-quality roughages on non-arable lands into human-edible foods.3
Despite the numerous contributions made globally by livestock, significant opportunity exists in many countries to vastly improve the efficiency of the production of animal-source foods while simultaneously reducing the environmental impact of livestock production.4 One of the proven ways in which to achieve those needed changes is through the wise use of reproductive technologies such as artificial insemination and embryo transfer. Artificial insemination (AI) and embryo transfer (ET) are reproductive technologies that are used to facilitate the genetic improvement of livestock. With AI, genetically superior males produce a larger number of highly productive offspring than would occur with conventional breeding programs. Similarly, with ET genetically superior females produce a larger number of highly productive offspring than would occur with conventional breeding programs.
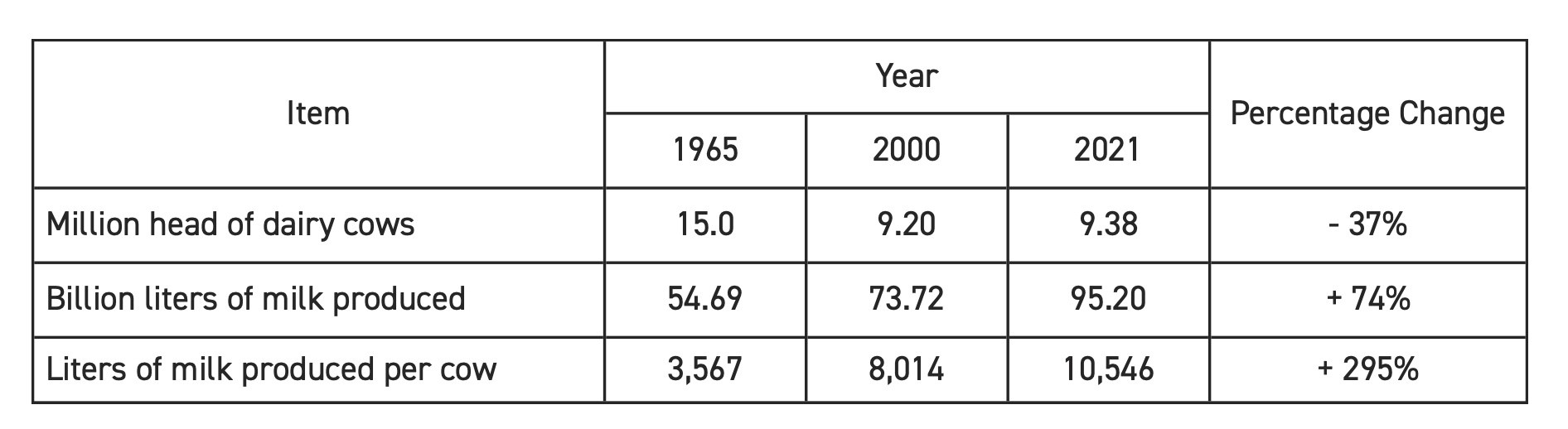
The under-harnessed power of reproductive technologies
To understand the power of these reproductive technologies, one needs only to look at the U.S. dairy cattle industry over the past several decades. Table 1 depicts changes that resulted after the widespread adoption of AI (beginning in 1965) and the widespread adoption of ET (starting in 2000). Between 1965 and 2021, the number of dairy cows in the U.S. dropped by 37%, the national milk supply increased by 74%, and the productivity per cow increased by 295%.
The beautiful part of the genetic change achieved with AI and ET is that this genetic improvement also improved the sustainability of the U.S. dairy cattle industry; improved genetics and improved animal management reduced the carbon footprint of the U.S. dairy cattle industry. One study 5 revealed that the carbon footprint of the 2007 U.S. dairy cattle industry was only 37% of the carbon footprint of the 1944 U.S. dairy cattle industry. Per liter of milk produced, the 2007 U.S. dairy cattle industry used only 10% of the land, 21% of animals, 23% of feedstuffs, and 35% of the water of the 1944 industry. These changes are illustrated in Figure 1.
A subsequent study6 compared the 2017 and 2007 U.S. dairy cattle industries. Results showed that, per unit of milk produced, the 2017 industry used only 79.2% of the land, 74.8% of animals, 82.7% of feedstuffs, and 69.5% of water. Outputs per unit of milk produced were only 79.4% of manure and 80.9% of methane (a greenhouse gas). This model for efficient milk production, if adopted more broadly on a global scale, would enhance the sustainability of milk production. This model could also be adapted for use in other livestock species.
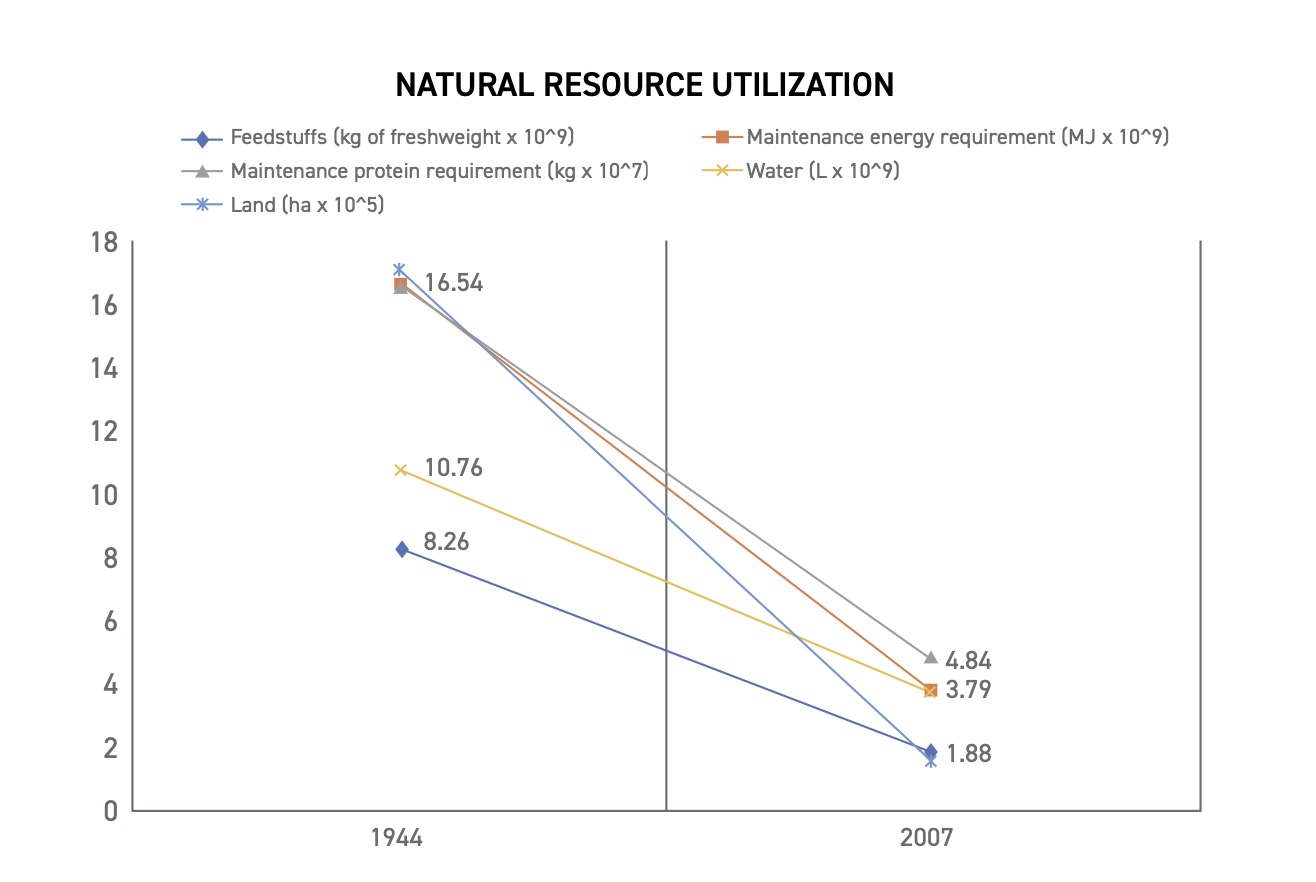
Sexed sperm cells for AI
In addition to conventional AI and ET, other reproductive technologies exist that can be used to further enhance sustainable livestock production. One of these technologies is sperm sexing.7 Sperm sexing is a technology that results in the physical separation of Y chromosome-bearing sperm from X chromosome-bearing sperm. When fertilizing a mammalian egg (which contains a single X chromosome), a Y chromosome-bearing sperm would produce a male embryo whereas an X chromosome-bearing sperm would produce a female embryo. By performing AI with sexed sperm cells, it is possible to pre-determining the genetic sex of a newborn animal with an accuracy between 85% and 95%. Beef cattle farmers, for example, often prefer that their cows give birth to male calves because male calves are more muscular, grow more quickly, and are more feed efficient than female calves. On the other hand, dairy cattle farmers often prefer female calves because only females can become pregnant, give birth, and subsequently produce milk.
One of the interesting applications of sexed sperm technology is called “beef on dairy”.8 Male dairy calves typically have low economic value because they lack the same genetic characteristics as male calves from breeds of cattle that have been genetically selected for meat production. In the “beef on dairy” approach, the highest-producing dairy females in a herd undergo AI with “female” semen (approximately 90% X-chromosome-bearing sperm cells) from a dairy breed bull to generate female calves that will enter the breeding herd after reaching sexual maturity. All remaining females in the milking herd undergo AI using “male” semen (approximately 85% Y chromosome-bearing sperm) from a beef breed bull that has been genetically selected for meat quality. This breeding approach allows the best milking females to produce daughters with high genetic potential for milk production and the below- average milking females to produce sons with higher genetic potential for meat production.
ET for better livestock
As stated previously, embryo transfer (ET) enables genetically superior females to produce a larger number of highly productive offspring than would occur with conventional breeding programs. The world’s first calf produced by ET was born on December 19, 1950 in Wisconsin, U.S.A.9, and today more than 1 million bovine embryos are transferred each year across the globe.10 The power of ET has been demonstrated not only by the creation of cows exhibiting record milk production (e.g., Ever-Green-View-My-Gold- ET who produced 35,218 kg of milk, 905 kg of butterfat, and 934 kg of protein in a single lactation), but also by the creation of bulls who are subsequently used as AI sires to generate highly productive offspring.11
Embryos may be produced in one of two ways: in vivo (in the living cow) or in vitro (in the laboratory). The in vivo derived (IVD) embryos are produced through a process called MOET (multiple ovulation and embryo transfer). During the MOET procedure, the genetically elite females (called “donors”) whose eggs will be fertilized to create embryos receive a series of booster shots to bolster their blood concentrations of the naturally occurring hormone FSH (follicle stimulating hormone). These booster shots help the cow produce multiple embryos. In contrast, the in vitro produced (IVP) embryos are created by harvesting immature eggs directly from the ovarian follicles of genetically superior cows using a procedure called ovum pick-up. Harvested eggs are subsequently matured and fertilized in the laboratory. The fertilized eggs (embryos) are grown in an incubator in the laboratory until the time of ET. The production of IVP embryos in livestock is quite similar to the procedures used in human infertility clinics to help couples produce children.
There are several advantages to IVP embryos. Firstly, more embryos can per produced per donor female per year due to the increased frequency with which the procedure can be performed (compared with MOET). Secondly, one straw of semen can be subdivided to fertilize eggs from numerous donor females (versus only one female with AI). Thirdly, immature eggs can be recovered, and embryos can be produced from females during early pregnancy and in the first few weeks after giving birth (something that cannot happen with conventional breeding).
Genomic testing technology
One genetic tool that has enhanced the results obtainable by using AI, ET, and/or in vitro embryo production is called genomic testing. With this genetic technology12, the genetic makeup of an animal is examined to locate short pieces of DNA where there is only one of the DNA building blocks (called nucleotides) that differs across animals (Figure 2). This difference is called a single nucleotide polymorphism (SNP), and animal geneticists have statistically correlated each SNP with animal performance. Animals can be genetically selected for the SNP which leads to greater productivity.
To identify SNPs, DNA can be extracted from a hair follicle or small drop of blood and then loaded onto an SNP chip (also called DNA microarray) where thousands of SNPs are simultaneously tested (e.g., a 50K SNP chip tests 50,000 different DNA fragments for SNPs).
The genetic information acquired from the SNP chip is used to calculate genomically enhanced estimated breeding values (EBVs) that are used when selecting ET donors and AI bulls. Figure 3 illustrates the rate of genetic improvement in one trait in dairy cattle called Net Merit Dollars PTA (predicted transmitting ability). After the implementation of genomic selection in 2009, the rate at which genetic improvement was being made doubled. Genomic testing has also been highly useful to identify carriers of recessive (hidden) genes impacting fertility and animal health.
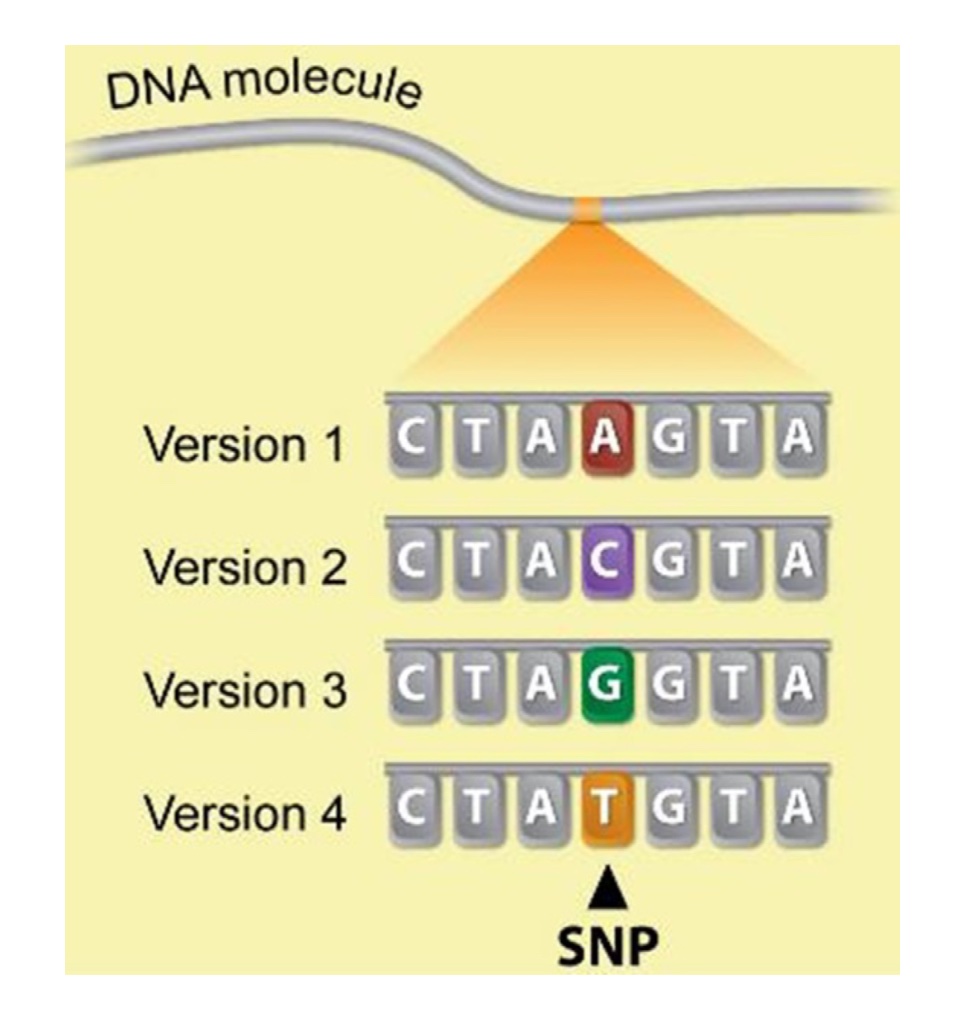
Genome editing of livestock
A relatively new technology that is in use by major livestock breeding companies is called genome editing.13 Genome editing uses one of several different naturally occurring enzyme systems (e.g., CRISPR/Cas 9; TALENS; ZFN) to induce an intentional genomic alteration (IGA) in a recently fertilized egg using a naturally occurring gene. Compared with traditional breeding programs, genome editing offers three distinct advantages: 1) very precise genetic changes can be made in an animal (versus introducing tens of thousands of genes at a time), 2) genetic change occurs more quickly (in as little as one generation), and 3) animals with a more desirable combination of genes than is possible through conventional breeding can be created.
Genome-edited cattle14 have already been produced with the polled gene which eliminates the need to remove horns from those cattle, the myostatin gene for increased muscling, and the SLICK gene for increased heat tolerance.15 On March 7, 2022, the U.S. Food and Drug Administration (FDA) announced16 that, after a thorough safety review, genome-edited cattle possessing the SLICK locus were declared as “Low-Risk” with respect to food safety. This determination was made in part because the genome editing procedure used a gene that naturally occurs in some breeds of cattle, and it resulted in an animal with an equivalent genotype to one that could be achieved over time using conventional breeding. A study of genome-edited cattle possessing the polled gene revealed that genome-edited cattle did not differ from non-edited control cattle with respect to growth, health, development, meat composition, or milk composition.17
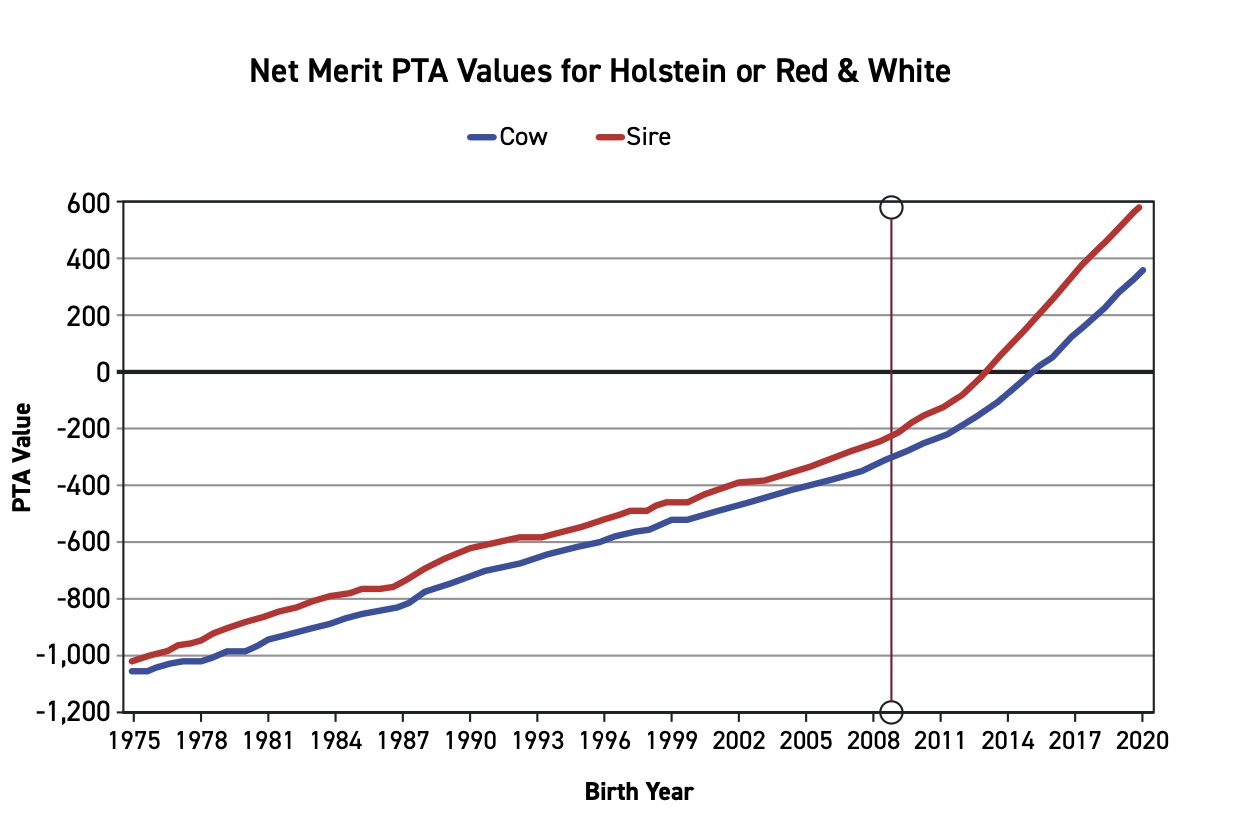
The collection of reproductive and genetic technologies described herein provides an avenue through which the sustainable production of animal-source foods can be achieved. These technologies represent climate-smart livestock production practices that are necessary for livestock farmers to be good stewards of natural resources during the production of animal-source foods that are such an important component of a healthy, well-balanced human diet.
Literature Cited
-
- White RR and MB Hall. 2017. Nutritional and greenhouse gas impacts of removing animals from US agriculture. PNAS (USA) 114 (48): E10301-E10308, https://doi.org/10.1073/pnas.1707322114
- FAO, IFAD, UNICEF, WFP and WHO. 2021. The State of Food Security and Nutrition in the World 2021. Transforming food systems for food security, improved nutrition and affordable healthy diets for all. Rome, FAO. https://doi.org/10.4060/cb4474en
- Mottet A, C de Haan, A Falcucci, G Tempio, C Opio, and P Gerber. 2017. Livestock: On our plates or eating at our table? A new analysis of the feed/food debate. Global Food Security 14:1–8, https://doi.org/10.1016/j.gfs.2017.01.001
- World Bank. 2022. Moving Towards Sustainability: The Livestock Sector and the World Bank. https://www.worldbank.org/en/topic/agriculture/brief/moving-towards-sustainability-the-livestock-sector-and-the-world-bank (accessed 27 November 2022)
- Capper JL, RA Cady, and DE Bauman. 2009. The environmental impact of dairy production: 1944 compared with 2007. Journal of Animal Science. 87(6):2160–2167, https://doi.org/10.2527/jas.2009-1781
- Capper JL and RA Cady. 2020. The effects of improved performance in the U.S. dairy cattle industry on environmental impacts between 2007 and 2017. Journal of Animal Science, 98(1);skz291, https://doi.org/10.1093/jas/skz291
- Vishwanath R. and J Moreno. 2018. Semen sexing – current state of the art with emphasis on bovine species. Animal 12(S1):S85-S96. https://doi.org/10.1017/S1751731118000496
- Cabrera VE. 2022. Economics of using beef semen on dairy herds. JDS Communications 3(2):147-151, https://doi.org/10.3168/jdsc.2021-0155
- Willett EL, WG Black, LE Casida, WH Stone, and PJ Buckner. 1951. Successful transplantation of a fertilized bovine ovum. Science 113(2931):247. https://doi.org/10.1126/science.113.2931.247.a
- Viana JHM. 2021. 2020 Statistics of embryo production and transfer in domestic farm animals. Embryo Technology Newsletter 39(4):24-38. https://www.iets.org/Portals/0/ Documents/Public/Committees/DRC/IETS_Data_Retrieval_ Report_2020.pdf
- Sommer MM and CR Youngs. 2016. Impact of embryo transfer technology on the production of artificial insemination sires for the U.S. dairy cattle industry. Animal Industry Report, Iowa State University, AS 662, ASL R3072, https://doi.org/10.31274/ans_air-180814-202
- Georges M, C Charlier, and B Hayes. 2019. Harnessing genomic information for livestock improvement. Nature Review Genetics 20:135–156, https://doi.org/10.1038/s41576-018-0082-2
- Menchaca A. 2021. Sustainable food production: The contribution of genome editing in livestock. Sustainability 13:6788. https://doi.org/10.3390/su13126788
- Bishop TF, and AL Van Eenennaam. 2020. Genome editing approaches to augment livestock breeding programs. Journal of Experimental Biology 223(Pt Suppl 1):jeb207159. https://www.doi.org/10.1242/jeb.207159
- Rodriguez-Villamil P, FL Ongaratto, JR Bostrom, S Larson, and T Sonstegard. 2021. Generation of SLICK beef cattle by embryo microinjection: A case report. Reproduction, Fertility and Development 33:114, https://doi.org/10.1071/RDv33n2Ab13
- United States Food and Drug Administration. 2022. FDA makes low-risk determination for marketing of products from genome-edited beef cattle after safety review. https://www.fda.gov/news-events/press-announcements/ fda-makes-low-risk-determination-marketing-products- genome-edited-beef-cattle-after-safety-review (accessed 27 November 2022).
- Trott JF, AE Young, BR McNabb, X Yang, TF Bishop, and AL Van Eenennaam. 2022. Animal health and food safety analyses of six offspring of a genome-edited hornless bull. GEN Biotechnology 1(2):192-206, http://doi.org/10.1089/genbio.2022.0008
Read and download this full eBook ‘Reproductive technologies for sustainable livestock production’ here