Healthy soil, the foundation of thriving ecosystems, is a complex dance between physical, chemical, and biological processes. While soil biology plays a crucial role, a single test doesn’t tell the whole story. This article delves deeper, exploring the factors that contribute to healthy soil and the various approaches used to assess it
What is healthy soil?
A healthy soil has biological processes in balance with physical and chemical processes (Fig 1). In agricultural soils for example, soil type has a significant influence on soil chemical and physical fertility, but healthy soils also have a strong contribution of biological processes associated with soil organisms, plant diversity, environmental conditions and the climate. Healthy soil is underpinned by land management practices that align with the principles of soil health 1. This approach addresses fertiliser practices, including placement, scheduling, and application procedures for nutrients, as well as enhancing soil biological fertility. While the contributions of soil organisms are paramount to the health of soil, individual soil biology tests are not necessarily useful indicators of healthy soil.
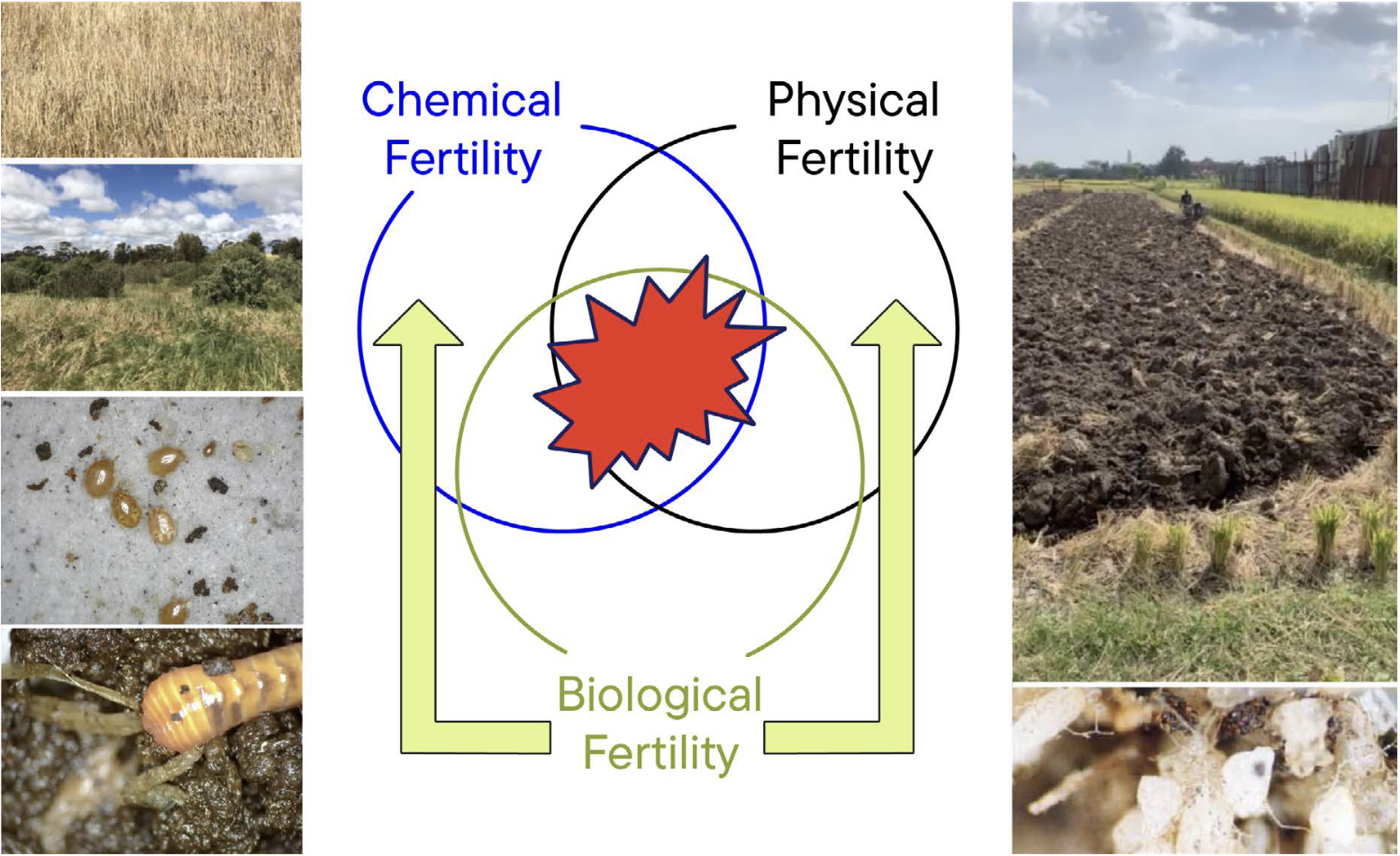
Over time, agricultural land management has evolved from use of natural, pre-industrial nutrient sources to widespread use of refined chemical fertilisers for overcoming soil constraints to plant production and to increase food and fibre production to levels that support population growth. During these transitional developments, the introduction of microbial inoculants (especially symbiotic nitrogen-fixing bacteria) was an important advance, as has been the use of various natural pest and disease control agents. Attention to sustainable land use practices (such as no-till and other conservation practices) and integrated pest management have helped contain serious land degradation and place greater emphasis on the importance of sustainable agriculture. Today, there is widespread interest in the use of biological amendments, including plant biostimulants (living and non-living), microbial inoculants and soil conditioners that promote plant production for human and animal health needs. More recently, this has evolved into a significant industry with a search for products and processes of biological origin that have the potential to enhance soil quality and contribute to building healthy soil.
Significance of soil type for healthy soil
Soils are primarily composed of mineral fractions (sand, silt and clay with an array of organic fractions mainly of dead plant matter and a smaller fraction of living organisms, including microbial biomass (Fig 2)). The relative proportions of sand, silt, and clay dictate the potential of soil to retain water and nutrients and influence its capacity to store carbon. Although the roots of many plants can penetrate to considerable depths below the soil surface, the depth of the soil profile suitable for agricultural production is influenced by the nature of the parent rock and either the extent and duration of time over which weathering has occurred or the extent of deposition of weathered material (via water and wind erosion). Consequently, soils worldwide have vastly different characteristics, which generally constrain their capacity to support agricultural production in their natural state and require management, including the use of soil amendments, to enhance their productive capability.
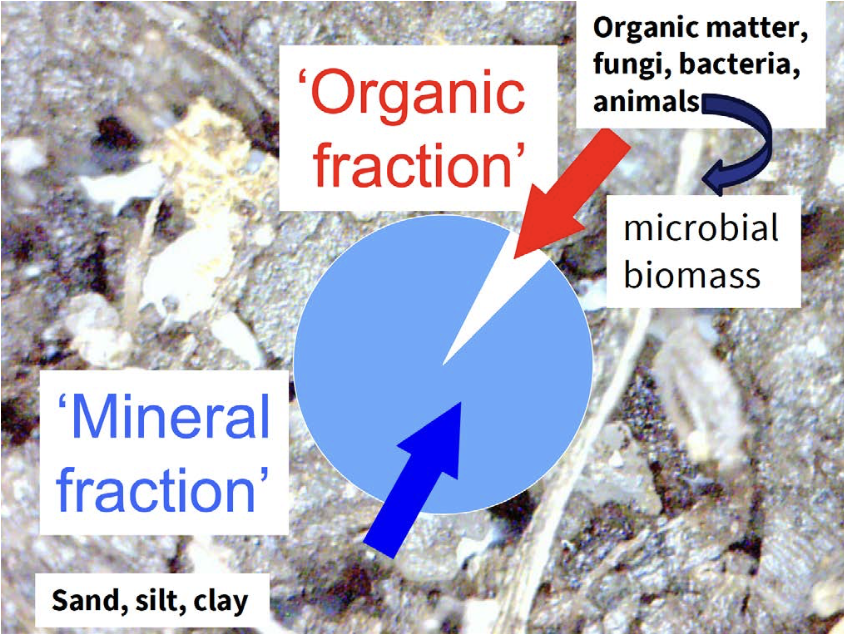
Significance of biodiversity for healthy soil
Locally, the abundance and diversity of soil organisms in agricultural soils are influenced by soil type, environmental conditions and many other factors, including plant species diversity and land management practices. These organisms provide essential ecosystem services, including at a landscape scale, via their involvement in the decomposition of soil organic matter and nutrient cycling, symbiotic associations with roots (e.g. mycorrhizal associations 3) building and sustaining soil structure, and sequestration of soil carbon. The diversity of soil organisms 4 ranges from very small (bacteria and fungi) to micro-fauna, meso-fauna, and macro fauna; they interact to cycle nutrients from plant residues and biological amendments. These processes have a significant capacity to complement nutrient supply from seasonal applications of inorganic or organic fertilisers, but their contributions can be overridden by excessive use of soil amendments, especially when nutrients are applied at levels beyond plant requirements. In addition, a healthy soil community has potential to control the development of plant disease in combination with wise land use practices and rotation of plant species. When contaminated materials are applied to soil (which could include contaminants in composted materials) or are stored in soil as waste, soil biological processes can be both beneficial and detrimental, depending on the nature and quantity of the contamination and where and how the waste is located in the landscape.
Significance of plant diversity for healthy soil
Many soil organisms interact closely with plants, including in and around their roots. Some form symbiotic associations, some cause root disease, and others prioritise their activities around the exudates, which come from roots and participate in plant nutrient uptake processes. Root growth alters the relative abundance of soil organisms, and this occurs in sequence throughout the plant growth cycle. Some soil organisms influence soil structure, especially soil aggregate stability and soil penetrability, which can alter root growth patterns.
Soil organic matter provides sources of energy and carbon for the majority of soil organisms. Most soil organic matter is derived from plants – both roots and above-ground plant residues. Although some groups of soil organisms do not derive their carbon and energy requirements from soil organic matter, they can be indirectly influenced by organic matter degradation processes. For example, grazing can significantly influence the release of root exudates as a source of carbon for use by organisms associated with roots. Overgrazing can leave the soil surface exposed to erosion by wind and water. Similarly, poor crop residue management can reduce the availability of important sources of soil carbon and leave the soil surface exposed. Advances in knowledge of good grazing practices and crop residue retention (e.g. less set stocking and reduced tillage) have led to more effective organic matter management. Overall, the interconnectivity of communities of soil organisms and plants is fundamental to soil health, with an emphasis on soil carbon (Fig 3).
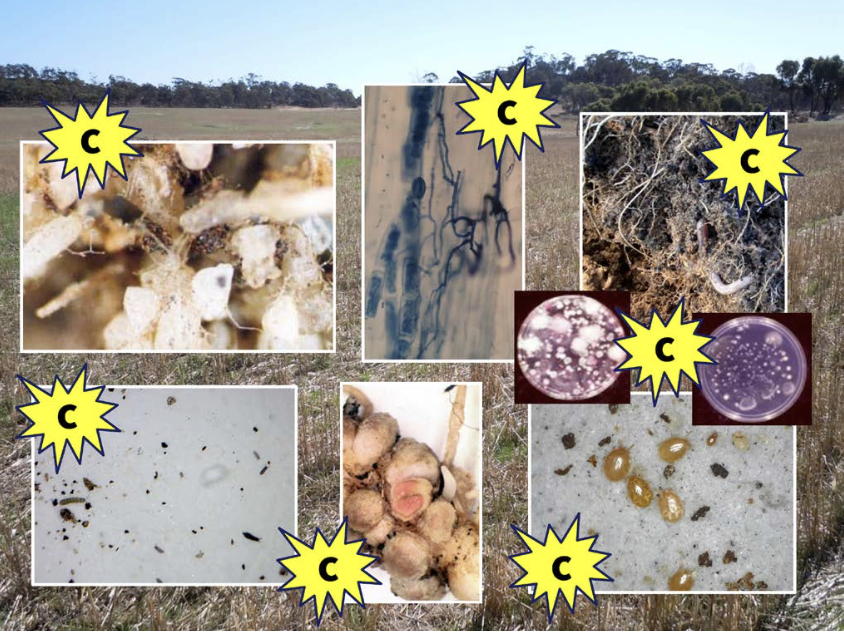
Retention of carbon in soil is fundamental to sustainable agriculture but the extent to which this occurs depends on the soil type and the degree and form of disturbance imposed by agricultural practices – this is because they are linked to the capacity of the soil to protect soil organic matter from being degraded by soil organisms. Thus, while soil organisms provide important ecosystem services, they are also responsible for the loss of soil carbon. This may appear to be a contradiction – but continuous activity of most soil organisms is not required in healthy soil. Protection of soil carbon is also an important soil process to which some organisms contribute (e.g. mycorrhizal fungi that form mycelial networks and bacteria that produce sticky polysaccharides while degrading plant organic matter). Nevertheless, many beneficial soil biological processes can be unnecessarily constrained by agricultural management practices, including continuous cropping and excessive nutrient application via fertilisers or effluent. Although these practices result in high levels of agricultural production, such production is not directly linked to soil health.
Significance of climate for healthy soil
Climate can influence soil health by altering the environment of soil communities – via indirect impacts on plants and via direct impacts on processes carried out by soil organisms. This relates to changes in rainfall (and hence soil moisture – less and more), water and wind erosion, air and soil temperatures, greenhouse gas concentrations, and the length of the growing season. These climate-related factors can also have potential impacts on plant growth (including roots), with the need for changes in plant varieties to adapt to climate change, which further influences soil communities.
Can soil biology tests indicate whether soil is healthy?
Many tests are available for identifying and quantifying the abundance of soil organisms or the processes in which soil organisms participate. However, there are two important questions in relation to soil health:
Can one or more soil biology tests predict whether the soil has the potential to be healthy given current or proposed land management practices?
There is a strong relationship between soil carbon and soil productivity (Dang et al. 2024) 5. Soil carbon is linked to soil fertility via plant shoot growth (e.g. via nutrient supply), root growth (e.g. via nutrient supply and soil physical and chemical conditions), and soil biological activity (e.g. via the involvement of soil organisms in nutrient cycling, symbiotic associations with plants and soil structural integrity). These are diverse processes and require a range of approaches for assessment. However a soil biological measurement is not necessarily an indicator of the state of the soil. Soils differ in their potential for productivity, therefore biological measurements need to be considered in the local context, and in terms of what they might predict about current or future soil conditions.
Quantification of soil biodiversity
Quantification of soil biodiversity requires assessment of which organisms are present. It is well known that many soil organisms cannot be isolated from soil and identified, but a range of molecular technologies have significantly advanced understanding of the diversity of soil organisms 4. Both direct and indirect approaches enable clarification of the complexity of soil communities. This may include specific organisms such as rhizobia, earthworms, mycorrhizal fungi, root pathogens (e.g. bacteria, fungi, some nematodes), communities of fungi and bacteria (e.g. molecular-based assessment of groups of organisms), and groups of soil animals (e.g. mites, nematodes, earthworms). It is also possible to estimate the relative dominance of bacteria and fungi, and to use identifier molecules (e.g. phospholipid fatty acid (PFLA) signatures) that are specific to groups of organisms such as fungi for quantification purposes. Although it is possible to follow changes in the abundance of organisms over time or how they change in abundance relative to similar or different organisms at the same location, the time required to do this and the cost involved can be considerable.
Quantification of soil biological activity
Quantification of soil biological activity and potential activity provide opportunities to predict how land management practices are likely to influence soil conditions that are important for soil health. In contrast to quantification of soil biodiversity, there is an opportunity to measure some activities of soil organisms, usually collectively for groups involved in the same processes. For example, soil respiration can be measured to give an overall estimation of activity of soil organisms at a point in time. This is most likely to reflect nutrient cycling activities during degradation of soil organic matter. Changes in the concentrations of forms of nitrogen in soil can reflect nitrification (ammonium oxidised to nitrate) or greenhouse gas release can reflect denitrification (loss of soil nitrogen as nitrous oxides). Enzyme activities can be used to track involvement of bacteria in nutrient transformations. Molecular-based analyses can be used to estimate gene function or potential gene function.
Soil collection for soil biology tests
A number of issues need to be considered prior to sampling soil to quantify soil organisms or the soil processes they are involved in. These include (i) what to sample, (ii) when to sample, (iii) where to sample, (iv) how many replicates are needed, and (v) how often to sample.
First, given the extensive diversity of soil organisms, and cost involved in analyses, there needs to be a specific reason for embarking on a program of soil biology tests. Sampling may be part of ongoing monitoring to determine how the soil biological community is influenced by land management practices. It may be used to identify the presence of a prominent root pathogen or to confirm the absence of symbiotic nitrogen-fixing bacteria in legumes. Other tests may involve descriptions of bacterial or fungal communities using molecular assays of whole soil communities. Identifying what will be sampled is the first step. The second step is to consider seasonal variation and, therefore, the most appropriate time of sampling. The third step requires an understanding of where the organisms are located in the soil profile and whether multiple soil sampling depths are required. The fourth step addresses variability in the data to be collected because it will be used to determine the number of replicate samples (either collected separately or bulked) so that important information is not overshadowed by a very high level of variation in the sensitivity of the test methodology or in the distribution of organisms or processes in the soil being measured. Finally, it is necessary to consider whether ongoing monitoring is necessary or one-off samples are adequate. This is particularly important because data that are fairly stable over time do not need to be collected as frequently as data that are more dynamic. Again, decisions about samping depend on the reason for taking the soil measurements.
Other soil sampling considerations can be highlighted by the following examples: quantification of rhizobia (their presence in root nodules on legumes or in soil) can provide an indication of both the occurrence and function of these symbiotic bacteria. Quantification of mycorrhizal colonisation within roots can provide an indication of the occurrence of the fungi, but not how well they are involved in nutrient uptake or soil aggregation. Quantification of the presence of plant pathogens in soil may be useful for predicting the likelihood of root disease as well as the potential risk of not pre-empting a potential problem. Nematode community analysis can be used to compare the biological status of soils (Ritz and Trudgill 1999) 6.
Overall, there are many different forms of soil biology tests. Some are potentially useful for experimental work to understand the life strategies of soil organisms and how they respond to land management, environmental pressures or climate change. Other methods can be used as monitoring tools to enable an understanding of how farming systems influence soil biological fertility and vice versa. However, a plan that addresses a specific reason for soil testing is recommended so that the best value can be gained in terms of understanding responses to agricultural disturbances and their predictive value in decision-making.
The Soil Health Institute (Bagnall et al., 2023) 7 recently suggested a minimum suite of soil health indicators for North American agriculture as (i) Organic carbon concentration, (ii) Carbon mineralisation potential (Respiration), (iii) and Aggregate stability, (iv) Prediction of Available Water Holding Capacity. This suggestion was based on an extensive analysis of potential indicators at 124 long-term agricultural research sites.
In another study, Romero et al., (2024) 8 investigated the link between soil health and primary productivity across 588 sites from 27 countries in Europe for three major land uses: woodlands, grasslands and croplands. They selected seven variables for use in a Soil Health Index, which included: N-fixing bacteria, Mycorrhizal fungi, Soil organic carbon, Phosphorus content, Soil density (compaction), Microbial biomass and Pathogen richness. In this case, the importance of variables in a soil health index depended on the land use under investigation.
In summary, the health of soil is best considered locally. Soils which are formed on sites with a history of volcanic activity or other processes that restore or retain soil minerals will probably be more productive than highly weathered soils. Clayey soils can store more carbon than sandy soils. Although soil types differ in their productivity potential, they can all be managed to be as ‘healthy’ as possible. Indeed, the characterisation of healthy soil should focus on the best possible outcome for a particular location, soil type, topography, land use and climatic exposure. The use of combinations of measurements or indicators of soil health also needs to be locally specific, involve knowledge of the land manager, and include monitoring for understanding the impacts of land management practices. The principles of Soil Health 1 align with all soil types, topography, and climate outcomes and provide the potential to build a healthy soil environment for agricultural production that makes efficient use of fertilisers, soil amendments, and biostimulants and is both profitable and resilient.
Acknowledgements
The inaugural General Jeffery Soil Health Award which was presented to Lynette Abbott is gratefully acknowledged.
References
- Principles of Soil Health: Sustaining profitable agricultural production.
- Soil Health Characteristics
- Soil health: A role for arbuscular mycorrhizal
- Soil biodiversity is essential for building environmental resilience
- Dang et al. 2024 Updating soil organic carbon for wheat production with high yield and grain protein. Field Crops Research 317 (2024) 109549
- Ritz and Trudgill 1999 Utility of nematode community analysis as an integrated measure of the functional state of soils: perspectives and challenges. Plant and Soil 212: 1–11.
- Bagnall et al. 2023 A minimum suite of soil health indicators for North American agriculture. Soil Security 10 (2023) 100084
- Romero et al. (2024) Soil health is associated with higher primary productivity across Europe. Nature Ecology & Evolution