Q fever is an emerging problem in low and middle-income countries (LMIC) and, therefore, there is a need for improved vaccines, in the view of Dr. Tom McNeilly, Head of Disease Control at Moredun Research Institute
Q fever, caused by the gram-negative intracellular bacteria Coxiella burnetii, is an important and highly contagious zoonotic disease of global importance. C. burnetii is thought to have a global distribution, with the exception of New Zealand and Antarctica, and is a significant cause of both human and livestock disease in many Development Assistance Committee (DAC)-listed countries. In grazing livestock, which are the main reservoir of C. burnetii, the infection is predominantly asymptomatic but can cause reproductive problems, such as abortion, the birth of weak offspring or infertility. In humans, the disease usually presents as a non-specific febrile illness, pneumonia and/or hepatitis which is usually self-limiting; however, around 2-5% of human cases will develop persistent infections, including endocarditis and vascular infection (1). This non-specific nature of the disease in humans presents a significant problem for the diagnosis and treatment of the condition in humans, particularly in resource-limited settings.
Epidemiology in ODA recipient countries
Recently, a number of epidemiological studies have been performed in countries within sub-Saharan Africa, including Tanzania, Ethiopia and Kenya, all of which are on the DAC-list of Official development assistance (ODA) recipient countries. These studies indicate endemicity of C. burnetii in both humans and ruminant livestock in these countries, with seroprevalence of up to 32%, 20%, 31% and 54% for humans, sheep, cattle and goats respectively, and that contact with grazing livestock is associated with increased risk of human infection (2-5).
Furthermore, studies in resource-limited settings indicate that Q fever is a common and increasingly recognised cause of febrile illness and community-acquired pneumonia (6, 7). Indeed, a recent study of hospitalised patients in Northern Tanzania found that Q fever was a more common cause of febrile illness than malaria (8). In addition to the direct effects of Q fever infections on human health, infections of livestock cause a reduction in productivity resulting in significant socioeconomic and indirect negative health effects on humans, particularly for smallholder farmers in resource-limited settings. Together, this indicates that Q fever is a significant public health concern in sub-Saharan Africa and that contact with grazing livestock is a significant risk factor.
Q fever control
Despite the growing evidence of the importance of Q fever in resource limited settings, there is currently little active control of Q fever in sub-Saharan Africa, which is exemplified by a recent systematic review of C. burnetii epidemiology in Africa which failed to identify a single disease control study in the scientific literature (4). In addition to poor awareness of the disease, one of the major reasons for this lack of Q fever control in these countries is the lack of a safe, effective and affordable Q fever vaccine. Currently-available vaccines, while showing a useful degree of protection, are suboptimal as they need to be manufactured at high containment, meaning manufacture is both difficult and costly, and are highly reactogenic when administered to animals and humans.
At present, the only licenced vaccine for use in humans is available in Australia, where only people who have not been previously exposed to C. burnetii can be vaccinated. Therefore, the use of the vaccine requires a complex pre-screening process in which individuals require a skin and blood test (to look for cellular and antibody responses to the bacteria, respectively) prior to vaccination. The vaccine is currently limited to people, for example, slaughterhouse workers and veterinarians, at high risk of C. burnetii exposure.
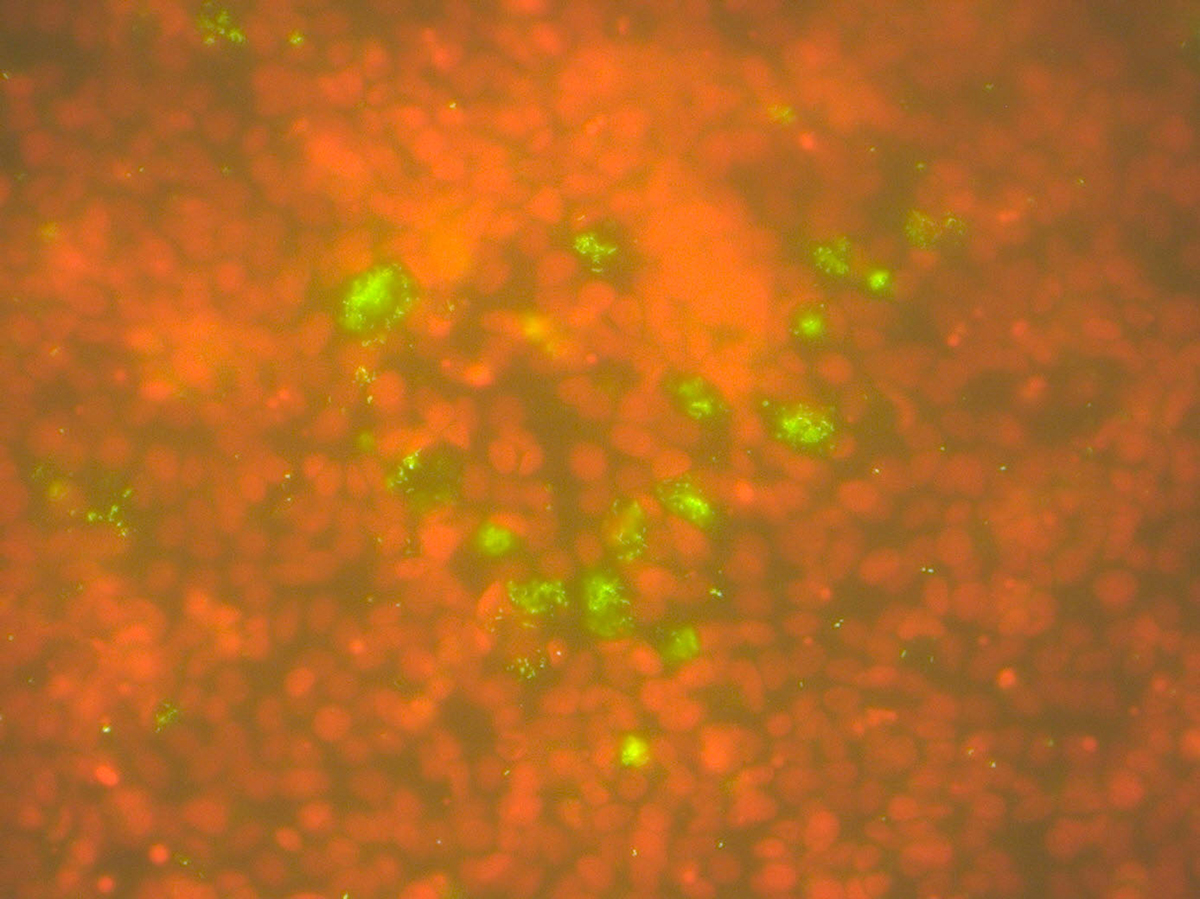
Clearly, there is a need to develop a safe, affordable and effective vaccine which can be deployed in resource-limited settings. Epidemiological data from a recent study in Kenya suggests that targeting the vaccination of grazing livestock going through markets may be an effective means of control (9).
Reducing the burden of disease in ruminant livestock, the main reservoir of infection, would provide significant economic and welfare benefits by:
1. Increasing livestock health and productivity resulting in socioeconomic improvements to livestock keepers;
2. Improving human health by reduction of zoonotic disease and;
3 Reducing costs associated with diagnosis and treatment of human Q fever cases.
Current status of Q fever vaccines
C. burnetii exists in phase I or phase II variants. Phase I C. burnetii, which is the virulent form of the bacteria, is isolated from natural sources and infections, plus it is a biosafety level 3 organism. In contrast, phase II C. burnetii is avirulent and is selected for after serial passage of phase I C. burnetii in embryonated eggs or tissue culture and is a biosafety level 2 organism (10). Currently licenced vaccines are based on formalin-inactivated phase I C. burnetii organisms and have proven efficacy in cattle, small ruminants and sheep.
Unfortunately, vaccines based on safer phase II C. burnetii are not effective (11). A key difference between phase I and phase II C. burnetii is the type of lipopolysaccharide (LPS) present, with a large ~26kb deletion in the genome of the phase II strain which encodes over 20 genes involved in LPS biosynthesis. This results in a severely truncated LPS that lacks the O antigen and some core sugars (10), suggesting that LPS is one of the main components in the commercial vaccines responsible for protection. However, protection associated with the phase I vaccine is not exclusively due to LPS as fractions of phase I C. burnetii enriched for LPS often induce lower levels of protection compared to whole phase I organisms (12, 13). Furthermore, an LPS based vaccine would still require manufacture at high containment, and may still induce injection site reactions.
In order to develop a safer Q fever vaccine, attempts have previously been made to develop subunit vaccines targeting key C. burnetii proteins (for example Type IV effector proteins which are thought to be promising antigens due to their key role in infection (14, 15) which would be safer and cheaper to manufacture and could be engineered to induce fewer side effects following immunisation. However, current approaches to subunit vaccine development have been severely hampered by a lack of knowledge of the appropriate antigens to target. As antibodies are thought to play a critical role in protection, previous attempts at identifying antigen targets have involved antibody screening of panels of C. burnetii proteins expressed as either E. coli recombinants (16) or using in-vitro translation systems (IVTS) (17); however, these approaches are costly, limited in their ability for repeatedly being probed, unpredictable in terms of ability to express individual proteins and inherently biased by what protein targets can be expressed. To date, despite these efforts, no commercial subunit vaccine for Q fever has been developed.
Current research on Q fever vaccines
Through a BBSRC and the UK Department of Health and Social Care funded CoxiMap project (BB/R019975/1) involving partners from the University of Copenhagen (Demark) and Wageningen University & Research (The Netherlands), peptide chip arrays representing the complete C. burnetii proteome are being used to identify the key linear B cell epitopes involved in protection associated with phase I C. burnetii vaccines. As this approach involves synthesised peptides rather than expressed bacterial proteins, it allows an unbiased interrogation of the whole bacterial proteome. By directly comparing epitopes detected by antibodies induced following immunisation of ruminants with protective phase I C. burnetii vaccines with those induced following immunisation with a “non-protective” phase II C. burnetii-based vaccine (11), protein antibody targets, i.e. C. burnetii antigens, which are associated with protection in the phase I vaccine will be identified. It is hoped that this approach, in which the antibody response of the protective phase I vaccine is characterised at the whole C. burnetii proteome level, will identify lead candidates for a novel safe and effective prototype subunit vaccine to control Q fever in both human and animal populations.
References
1.) Parker NR, Barralet JH, Bell AM. 2006. Q fever. Lancet 367:679-688.
2.) Njeru J, Henning K, Pletz MW, Heller R, Neubauer H. 2016. Q fever is an old and neglected zoonotic disease in Kenya: a systematic review. BMC public health 16:297.
3.) Gumi B, Firdessa R, Yamuah L, Sori T, Tolosa T, Aseffa A, Zinsstag J, Schelling E. 2013. Seroprevalence of Brucellosis and Q-Fever in Southeast Ethiopian Pastoral Livestock. Journal of veterinary science & medical diagnosis 2.
4.) Vanderburg S, Rubach MP, Halliday JE, Cleaveland S, Reddy EA, Crump JA. 2014. Epidemiology of Coxiella burnetii infection in Africa: a OneHealth systematic review. PLoS neglected tropical diseases 8:e2787.
5.) Njeru J, Henning K, Pletz MW, Heller R, Forstner C, Kariuki S, Fevre EM, Neubauer H. 2016. Febrile patients admitted to remote hospitals in Northeastern Kenya: seroprevalence, risk factors and a clinical prediction tool for Q-Fever. BMC infectious diseases 16:244.
6.) Prasad N, Murdoch DR, Reyburn H, Crump JA. 2015. Etiology of Severe Febrile Illness in Low- and Middle-Income Countries: A Systematic Review. PloS one 10:e0127962.
7.) Prabhu M, Nicholson WL, Roche AJ, Kersh GJ, Fitzpatrick KA, Oliver LD, Massung RF, Morrissey AB, Bartlett JA, Onyango JJ, Maro VP, Kinabo GD, Saganda W, Crump JA. 2011. Q fever, spotted fever group, and typhus group rickettsioses among hospitalized febrile patients in northern Tanzania. Clinical infectious diseases: an official publication of the Infectious Diseases Society of America 53:e8-15.
8.) Crump JA, Morrissey AB, Nicholson WL, Massung RF, Stoddard RA, Galloway RL, Ooi EE, Maro VP, Saganda W, Kinabo GD, Muiruri C, Bartlett JA. 2013. Etiology of severe non-malaria febrile illness in Northern Tanzania: a prospective cohort study. PLoS neglected tropical diseases 7:e2324.
9.) Wardrop NA, Thomas LF, Cook EA, de Glanville WA, Atkinson PM, Wamae CN, Fevre EM. 2016. The Sero-epidemiology of Coxiella burnetii in Humans and Cattle, Western Kenya: Evidence from a Cross-Sectional Study. PLoS neglected tropical diseases 10:e0005032.
10.) Hackstadt T, Peacock MG, Hitchcock PJ, Cole RL. 1985. Lipopolysaccharide variation in Coxiella burnetti: intrastrain heterogeneity in structure and antigenicity. Infection and immunity 48:359-365.
11.) Arricau-Bouvery N, Souriau A, Bodier C, Dufour P, Rousset E, Rodolakis A. 2005. Effect of vaccination with phase I and phase II Coxiella burnetii vaccines in pregnant goats. Vaccine 23:4392-4402.
12.) Waag DM, England MJ, Pitt ML. 1997. Comparative efficacy of a Coxiella burnetii chloroform:methanol residue (CMR) vaccine and a licensed cellular vaccine (Q-Vax) in rodents challenged by aerosol. Vaccine 15:1779-1783.
13.) Williams JC, Peacock MG, Waag DM, Kent G, England MJ, Nelson G, Stephenson EH. 1992. Vaccines against coxiellosis and Q fever. Development of a chloroform:methanol residue subunit of phase I Coxiella burnetti for the immunization of animals. Annals of the New York Academy of Sciences 653:88-111.
14.) Xiong X, Jiao J, Gregory AE, Wang P, Bi Y, Wang X, Jiang Y, Wen B, Portnoy DA, Samuel JE, Chen C. 2017. Identification of Coxiella burnetii CD8+ T-Cell Epitopes and Delivery by Attenuated Listeria monocytogenes as a Vaccine Vector in a C57BL/6 Mouse Model. The Journal of infectious diseases 215:1580-1589.
15.) Beare PA, Gilk SD, Larson CL, Hill J, Stead CM, Omsland A, Cockrell DC, Howe D, Voth DE, Heinzen RA. 2011. Dot/Icm type IVB secretion system requirements for Coxiella burnetii growth in human macrophages. mBio 2:e00175-00111.
16.) Wang X, Xiong X, Graves S, Stenos J, Wen B. 2013. Protein array of Coxiella burnetii probed with Q fever sera. Science China. Life sciences 56:453-459.
17.) Beare PA, Chen C, Bouman T, Pablo J, Unal B, Cockrell DC, Brown WC, Barbian KD, Porcella SF, Samuel JE, Felgner PL, Heinzen RA. 2008. Candidate antigens for Q fever serodiagnosis revealed by immunoscreening of a Coxiella burnetii protein microarray. Clinical and vaccine immunology: CVI 15:1771-1779.
Head of Disease Control
Moredun Research Institute
Tel: +(44)131 445 5111
Tom.McNeilly@moredun.ac.uk
https://www.moredun.org.uk/
www.twitter.com/MoredunComms
www.twitter.com/tnmcneilly
*Please note: This is a commercial profile