Professor Arpita Bose discusses how iron-based mediators can enhance electron uptake in electrotrophic bacteria, which are employed as biocatalysts in microbial electrosynthesis technologies
Climate change is no longer just a future concern; its impacts are being felt today. As these effects persist, the systems and structures foundational to human flourishing will suffer, leading to water shortages, food insecurity, economic destabilization, and more. This forces us to confront how our behavior today will impact the climate of tomorrow.
But how do we address these challenges? In the Bose lab, we are pursuing technologies that take inspiration from nature – specifically, the microbial world.
Converting CO2 into bioplastic using microbes
Our team at Washington University in St. Louis is working to solve the climate crisis by developing microbial electrosynthesis (MES) technologies. MES takes advantage of “electrotrophic” microbes that convert carbon feedstocks into valuable outputs using electricity. Some electrotrophs are useful tools for bioremediation, nitrogen fixation, and wastewater treatment; others can synthesize energy-rich multicarbon compounds (e.g., plastics and fuels).
In comparison to other MES systems, which often use chemoautotrophic microorganisms, our group is interested in photosynthetic bacteria such as Rhodopseudomonas palustris TIE-1. Organisms like TIE-1 hold great promise as sustainable biocatalysts due to their reliance on light and CO2 as energy and carbon sources, respectively. We previously demonstrated TIE-1’s ability to synthesize the bioplastic polyhydroxybutyrate (PHB) in an MES system. However, this study revealed that the key limitations for PHB production are poor extracellular electron uptake at the electrode surface and a low CO2 conversion efficiency.
Enhancing electron transfer with iron oxide-graphene nanoparticles
Because iron is the basis for redox mediators used in some electrochemical applications, and TIE-1 can interact with iron, we explored iron-based modifications to the electrodes. These modifications can enhance electron uptake and influence downstream cellular processes (e.g., CO2 fixation). We found that graphite rod electrodes coated with Prussian Blue (iron(III) hexacyanoferrate) enhanced electron uptake 3.8-fold compared to plain graphite electrodes.
Next, we modified a carbon felt (CF) electrode with a hydrothermally synthesized “reduced graphene oxide-magnetite nanoparticle” (rGO-MNP) nanocomposite (Figure 1). When used in a TIE-1 inoculated MES system, this novel electrode improved PHB production to 91.31 mgL-1 – a 20-fold increase compared to graphite electrodes and a 4.2-fold increase compared to plain CF electrodes. We also saw increases in electron uptake (5-fold higher than CF), faradaic efficiency (2-fold higher than CF), and cellular attachment to the CF/rGO-MNP electrode.
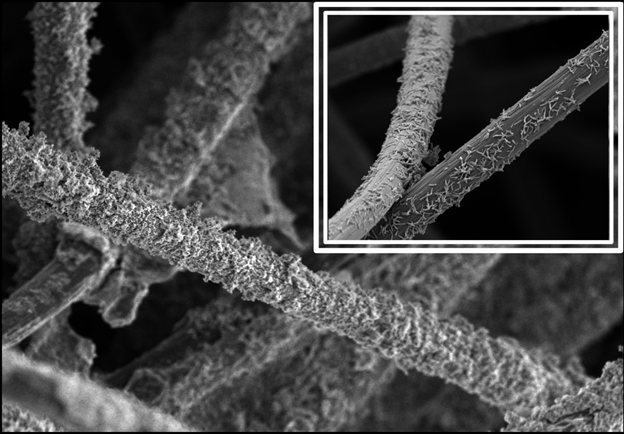
The experiments above show that we can improve MES output via electrode modifications using iron – the fourth most abundant element in Earth’s crust. Moreover, photosynthetic electrotrophs require only CO2, light, and electricity, of which the latter can be generated with solar energy. Together, we believe this lays the foundation for sustainable, scalable MES technologies for producing value-added products like bioplastic.
The future of electrode materials
Iron-based modifications are not the only possible route for improving electron transfer and product formation in MES. Other examples of electrode modifications include carbon nanotubes, 3D-graphene/carbon felt cathodes, indium nanoparticles, and more. Moreover, related technologies like microbial fuel cells have been the subject of extensive research in the realm of electrode materials, and lessons learned from these studies should be applied toward MES.
More broadly, investigators should keep in mind a variety of priorities (e.g., maximizing output, maximizing electron uptake, minimizing cost, biofilm attachment, etc.) when considering novel electrode materials and modifications. Because the balance of these priorities can vary in different contexts, it is important to explore diverse materials with varying strengths and weaknesses. This will afford a certain degree of flexibility and customization according to users’ needs as we move from the laboratory-scale to industrial-scale.
Authors: Karthikeyan Rengasamy, Tahina O. Ranaivoarisoa, Eric M. Conners, Arpita Bose