Manuka honey, made from tea trees, can help collagen scaffolds to heal by resisting bacterial infection and promoting bone growth
Bones in the face and skull can be affected by various conditions, including cleft palate defects, traumatic injuries, cancer, and bone loss from dentures.
There are ways of regenerating the missing tissue, such as bone replacements. However, these are vulnerable to bacterial infection.
45% of all hospital-contracted infections are the result of bone implants
It may be surprising, but bone implants account for 45% of all hospital-contracted infections. And this impedes healing in drastic ways.
Typically, these implants are made from biomaterials that contain extracellular matrix components. These are molecules providing structural support to cells.
It is commonplace for researchers to use metal implants or synthetic polymers to study bone defects and infections. Therefore, there is a gap in the understanding of how biomaterials behave in response to infection.
‘Imagine a metal versus something soft and porous’
“Imagine a metal versus something soft and porous that is made up of extracellular matrix components. They have very different characteristics,” said Marley Dewey, a former graduate student in the Harley lab and the first author of the paper.
“Using our scaffolds, this is the first paper to look at how these materials become infected.”
Using collagen scaffolds to study manuka honey properties
The researchers used collagen scaffolds to study whether manuka honey can be incorporated to inhibit the growth of two bacteria that commonly infect bone wounds: Pseudomonas aeruginosa and Staphylococcus aureus.
“At various conferences I saw researchers talk about how nature-derived materials can be used instead of antibiotics, circumventing the problem of antibiotic resistance. Additionally, when I looked at the literature, I found research that claimed manuka honey can be useful,” Dewey explains.
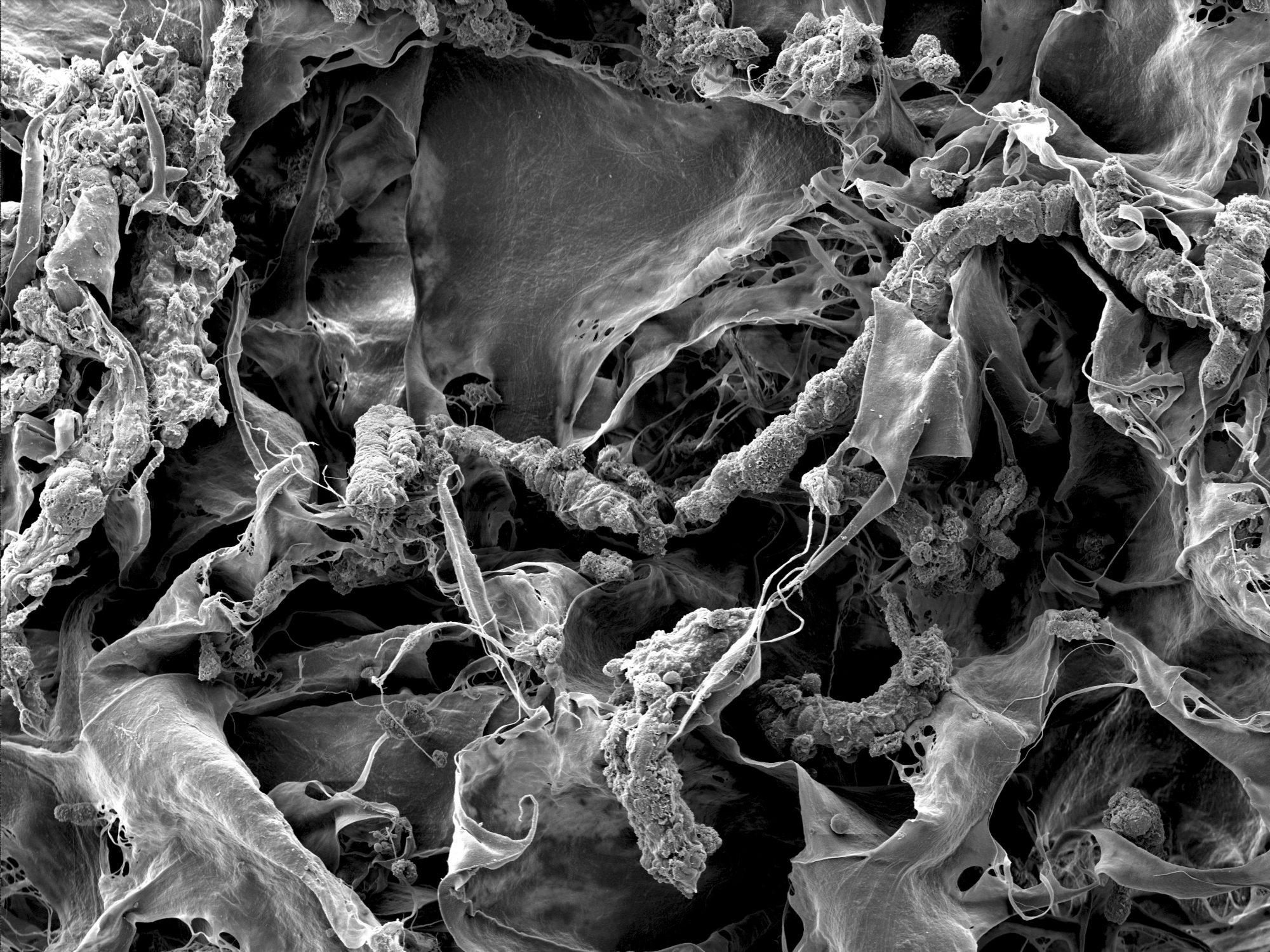
The team added the manuka honey into the scaffolds in two different ways: incorporation and soaking.
Their first method allowed them to add the honey to a collagen suspension and blended them together. The suspension was then used to create collagen scaffolds with honey embedded in their structure.
The second process differed in that they soaked the prepared collagen scaffolds in manuka honey for 40 hours. In both cases, they tested whether adding manuka honey affected bone growth and bacterial infection.
Testing different concentrations of manuka honey to find the perfect combination
“We focused on characterizing the mesenchymal stem cell response, which is important for bone repair since these cells differentiate to create bone,” Dewey said.
“We first tested different concentrations of honey to find the ideal concentration we need to use.”
It was discovered that when the collagen scaffolds were soaked in 5% honey, there was a higher mineral formation and osteoprotegerin production. This all points to increased bone production.
However, higher concentrations of honey actually led to decreasing bone health. It also became apparent that the soaked scaffolds worked better than the incorporated ones, although the reason is unclear.
Is the growth of P. aeruginosa and S. aureus inhibited by manuka honey?
In order to test whether the growth of P. aeruginosa and S. aureus is inhibited by manuka honey, the research team soaked paper discs in different concentrations of honey and added them to bacterial lawns.
They were very surprised to find that, in actual fact, one of the concentrations were able to prevent bacterial growth. Similarly, the honey-soaked scaffolds could not prevent bacterial growth, even though the bacteria could not attach to these surfaces.
“We measured bacterial growth with scanning electron microscopes; by using representative images, we were able to count the number of bacteria,” Dewey said.
“It was striking that we needed ten times the amount of antibiotics to kill the bacteria and some still survived,” Dewey adds. “It’s possible that it is easy to kill the bacteria when metal implants are used. However, our collagen scaffolds seem to offer a protective environment for bacteria, creating a big problem.”
What does the future hold for bacterial infection research?
“I want to create models that other people can use for implant infection. I’m also interested in understanding how bacteria interact with cells in the scaffolds and why they are so resistant to antibiotics in a scaffold structure,” Dewey concludes.
“This work was made possible with the help of the IGB themes,” said Brendan Harley (RBTE leader/EIRH), Robert W. Schaefer Professor of Chemical and Biomolecular Engineering. “Bringing in Alan Collins, a member of IGOH, was essential in the bacterial culture work. He helped us gain the expertise we needed to complete this study.”
Dewey hopes to continue working on how biomaterials can be used to prevent infection in a laboratory of her very own.