J.D. Huba from Syntek Technologies explains about modeling plasmasphere ducts as part of this focus on the plasmasphere, the extension of the earth’s ionosphere
The plasmasphere is the extension of the earth’s ionosphere into the inner magnetosphere along closed magnetic field lines. It is a relatively dense, cold plasma (ne > 102 cm−3 and T≲4 × 104 K) [Lemaire et al., 1998]. The conventional perception of the plasmasphere is that the electron density monotonically decreases with altitude and is relatively uniform, i.e., unstructured.
However, numerous studies of whistler wave propagation in the low- to mid-latitude ionosphere/plasmasphere system contrast ‘ducted’ versus ‘non-ducted’ wave propagation where ducts are nominally 10% to 20% increases or decreases in the electron density along the geomagnetic field over relatively small length scales (10s – 100s km) [Helliwell, 1965; Singh et al., 1998; Clilverd et al., 2008].
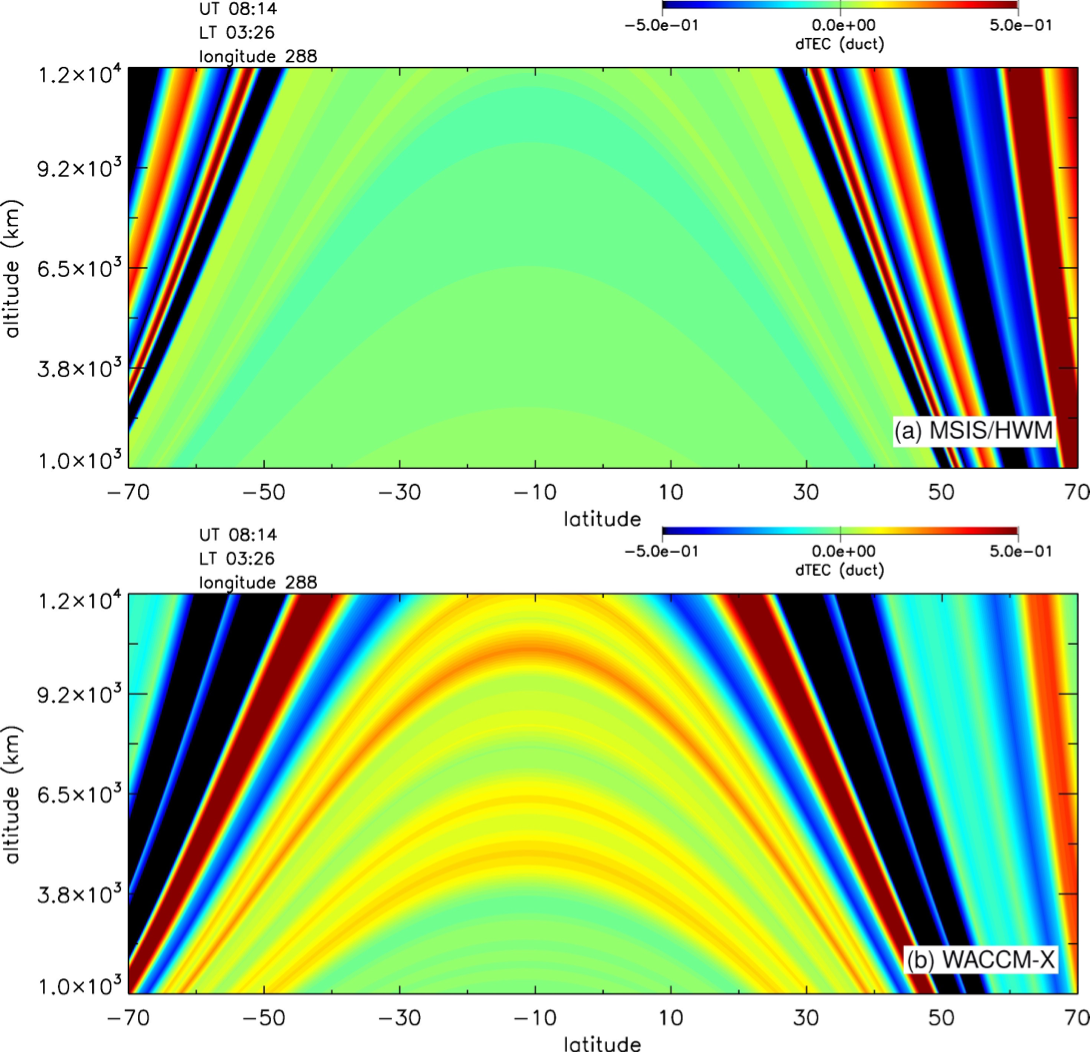
Plasmasphere ducts
Additionally, there have been observations of plasmaspheric ducts from radio-interferometric measurements [Jacobson et al., 1996; Loi et al., 2015; Helmboldt et al., 2020a, b] and density structures from satellite observations [Darrouzet et al., 2009] indicating that the inner plasmasphere is not spatially smooth as often characterized.
Plasmasphere ducts and whistler wave propagation are important because whistler waves interact with radiation belt electrons and scatter high-energy electrons into the lower atmosphere, where they are absorbed. This impacts the naturally occurring Van Allen radiation belts and man-made radiation belts [Gombosi et al., 2017].
Several theories have been suggested for the cause of plasmasphere ducts that rely on plasma instabilities occurring in the E region ionosphere [Cole, 1971; Perkins, 1973; Cosgrove and Tsunoda, 2002]. However, these instabilities only occur at night and cannot explain daytime duct formation.
We have recently shown that atmospheric gravity waves can generate plasma ducts in the plasmasphere using the coupled SAMI3/WACCM-X model [Huba and Liu, 2020]. Physically, gravity waves perturb the zonal and meridional neutral winds, which affect the plasma motion and electric field. Since the magnetic field lines are equipotentials, the perturbed electric field maps into the plasmasphere and can generate ducts.
An example of duct formation is shown in Fig. 1, which is a contour plot of the differential total electron content along the geomagnetic field (dTECduct) at longitude 288° and time 08:14 UT (corresponds to 03:26 LT). The top panel uses the empirical thermosphere models NRLMSISE0 [Picone et al., 2002] and HWM14 [Drob et al., 2015] as inputs to SAMI3. The bottom panel uses the physics-based model WACCM-X as inputs to SAMI3. The distinction between these two models is that WACCM-X includes atmospheric gravity waves while the empirical models do not. In both cases, ducts occur at high latitudes (e.g., above 50° latitude and below 75° latitude) that are associated with the high latitude convection potential.
However, more important, there is a clear formation of ducts at mid-latitudes in the WACCM-X case that do not form in the MSIS/HWM case. In this case the ducts are in the altitude range of ~ 4 − 18 × 103 km (corresponds to L~1.6−3.5)and can persist for 0.5-2.0 hrs at a given latitude. These results are consistent with observations of ducted VLF wave propagation in this L shell range and ducted wave lifetimes [Singh et al., 1997; Clilverd et al., 2008].
Looking ahead: Mid-latitude plasma ducts
Thus, we have shown a direct coupling between atmospheric gravity waves and the development of plasmasphere ducts based on a global thermosphere/ ionosphere/ plasmasphere model for the first time. These ducts play a critical role in the propagation of whistler waves that can affect the earth’s Van Allen radiation belts. Future work will determine the dependence of mid-latitude plasma ducts on longitude, season and solar activity and direct comparisons with observations.
References
-
- Cole, K.D., Formation of _eld-aligned irregularities in the magnetosphere, J. Atmos. Terr. Phys., 33, 741, 1971.
- Cosgrove, R. B. and R.T. Tsunoda, A direction-dependent instability of sporadic-E layers in the nighttime midlatitude ionosphere, Geophys. Res. Lett., 29, 1864, https://doi.org/10.1029/2002GL014669, 2002.
- Clilverd, M. A., C. J. Rodger, R. Gamble, N. P. Meredith, M. Parrot, J.-J. Berthelier, 64 and N. R. Thomson, Ground-based transmitter signals observed from space: Ducted 65 or nonducted?, J. Geophys. Res., 113, A04211, https://doi.org/10.1029/2007JA012602, 2008.
- Darrouzet, F. et al., Plasmaspheric density structures and dynamics: Properties observed by the CLUSTER and IMAGE missions, Space Sci. Rev., 145, 55, 2009.
- Drob et al., An update to the Horizontal Wind Model (HWM): The quiet time thermosphere, Earth and Space Science, https://doi.org/10.1002/2014EA000089, 2015.
- Gombosi, T.I., D.N. Baker, A. Balogh, P.J. Erickson, J.D. Huba, and L.J. Lanzerotti, Anthropogenic space weather, Space Sci Rev, 212, 985, https://doi.org/10.1007/s11214-017-0357-5, 2017.
- Helliwell, R.A., Whistlers and related ionosphere phenomena, Stanford: Stanford University Press, 1965.
- Helmboldt, J.F., J.D. Haiducek, and T.E. Clarke, The properties and origins of corotating plasmaspheric irregularities as revealed through a new tomographic technique, J. Geophys. Research: Space Physics, 125, e2019JA027483. https://doi.org/10.1029/2019JA027483, 2020a.
- Helmboldt, J.F., The properties and origins of corotating plasmaspheric irregularities: Part II|Tomography with compact arrays of GPS receivers, J. Geophys. Research: Space Physics, 125, 125, e2020JA027858. https://doi.org/10.1029/2020JA027858, 2020b.
- Huba, J.D. and H.-L. Liu, Global modeling of equatorial spread F with SAMI3/WACCM-X, Geophys. Res. Lett., 47, e2020GL088258. https://doi.org/10.1029/2020GL088258 2020.
- Jacobson, A.R., G. Hoogeveen, R.C. Carlos, G. Wu, B.G. Fejer, and M.C. Kelley, Observations of inner plasmasphere irregularities with a satellite-beacon radio-interferometer array, J. Geophys. Res., 101, 19,665, 1996.
- Lemaire, J., Gringauz, K., Carpenter, D., and Bassolo, V., The Earth’s Plasmasphere (Cambridge Atmospheric and Space Science Series), Cambridge: Cambridge University Press, https://doi.org/10.1017/CBO9780511600098, 1998.
- Loi, S.T. et al., Real-time imaging of density ducts between the plasmasphere and ionosphere, Geophys. Res. Lett., 42, 3707, https://doi.org/10.1002/2015GL063699, 2015.
- Perkins, F., Spread F and ionospheric currents,
J. Geophys. Res., 78, 218, https://doi.org/10.1029/JA078i001p00218, 1973. - Picone, J.M., A.E. Hedin, D.P. Drob, and A.C. Aikin, NRLMSISE-00 empirical model of the atmosphere: Statistical comparisons and scientific issues, J. Geophys. Res.107, https://doi.org/10.1029/2002JA009430, 2002.
- Singh, R.P., A.K. Singh and D.K. Singh, Plasmaspheric parameters as determined from whistler spectrograms: a review, J. Atmos. Solar-Terres. Phys., 60,495, 1998.
Dr. Hanli Liu was a key collaborator on this research project, providing the WACCM-X data inputs.
This research has been funded, in part, by the National Science Foundation (AGS1931415) and the Air Force Office of Scientific Research (FA9550-22-C-0001).

This work is licensed under Creative Commons Attribution-NonCommercial-NoDerivatives 4.0 International.