Dr Alan Schechter of the Molecular Medicine Branch at the National Institutes of Health in Bethesda, Maryland and his colleagues discuss research during the last two decades that has revealed a second major pathway for Nitric Oxide formation in mammals
The relatively staid world of the biology of gases was awakened in 1985 with the realisation that nitric oxide (NO), a reactive radical, had important physiological effects as a strong vasodilator. Within a few years it was established that this molecule was found almost ubiquitously in animal, plant and other living forms and acted as a signaling molecule with very diverse functions. Indeed, NO previously of primary concern as an atmospheric pollutant, could be considered to be the fourth important biological gas, along with oxygen, carbon dioxide and carbon monoxide – it and CO being produced in very small quantities relative to O2 and CO2.
Studies of Nitric Oxide formation
Studies of NO since 1985 have resulted in a literature of several hundred thousand peer reviewed publications. Its best known functions in mammalian cells include, in addition to relaxing smooth muscle of arterioles, inhibiting many cell-cell interactions, platelet activation and blood clotting; a variety of anti-microbial effects; and acting as a neurotransmitter. The original paradigm for the formation of NO was based on the discovery of three families of nitric oxide synthase enzymes (NOS I, II, and III), initially associated with neuronal, macrophage and endothelial cells, but subsequently found to be widely distributed, which synthesize NO from arginine. Much research in the decade or two after the discovery of the importance of Nitric Oxide was focused on these enzymes and their mechanisms and inhibitors, with the still to be realised hope of basing the development of new therapeutics on inhibiting NO formation.
However, the major therapeutic success of NO has been its delivery as a gas for the treatment of various pulmonary causes of hypoxia, especially in newborn children. Ironically, this success has called attention to the fact that earlier work on NO often assumed that its presence, in normal or increased concentrations, was the cause of various pathophysiological states. The efficacy of inhaled NO and the failure of a number of trials of NOS inhibitors gradually led to a 180º shift in the field to a search for ways to increase NO as a potential therapy for a variety of diseases. We, in collaboration with other investigators especially Dr Mark Gladwin then at NIH, in 1998 initiated studies of NO administration in sickle cell disease patients to see if we could improve the flow of rigid sickle red cells through the arterioles of these patients.
Although the effects of NO administration, initially by inhalation and later via other means, did not result in robust therapies, these procedures led us to observations of the existence of arterial-venous gradients of nitrite levels in the blood of normal individuals as well as patients. We hypothesised that postulated endocrine-like transport of NO bioactivity to hypoxic regions might occur via nitrite ion reduction by partially deoxygenated red cells in these vascular beds.
Over several years we confirmed this hypothesis in multiple studies. Our subsequent work, and that of investigators at the Karolinska Institute, the William Harvey Research Institute and other centres, extended these concepts in many important ways, including demonstrating reduction of nitrate ions as well as nitrite ions. The current view of how these reductive pathways contribute, especially under greater or lesser hypoxia, to the more “classical” formation of NO by enzymatic pathways under high oxygen concentrations is shown in the accompanying Figure.
The conversions among nitrate and nitrite ions and NO in mammals by both reductive and oxidative pathways can be represented by a triangle which is a subset of the overall biological nitrogen metabolic pathways, which include N2, nitrous oxide (N2O) and ammonia (NH3), as first described by Dr V.P. Reutov. These pathways are crucial for atmospheric nitrogen fixation in nature by legumes and other organisms. Plants, bacteria and other microorganisms have enormous numbers of enzymes that have evolved for these purposes and have been extensively characterised but these pathways are only now being identified and studied in mammalian cells.
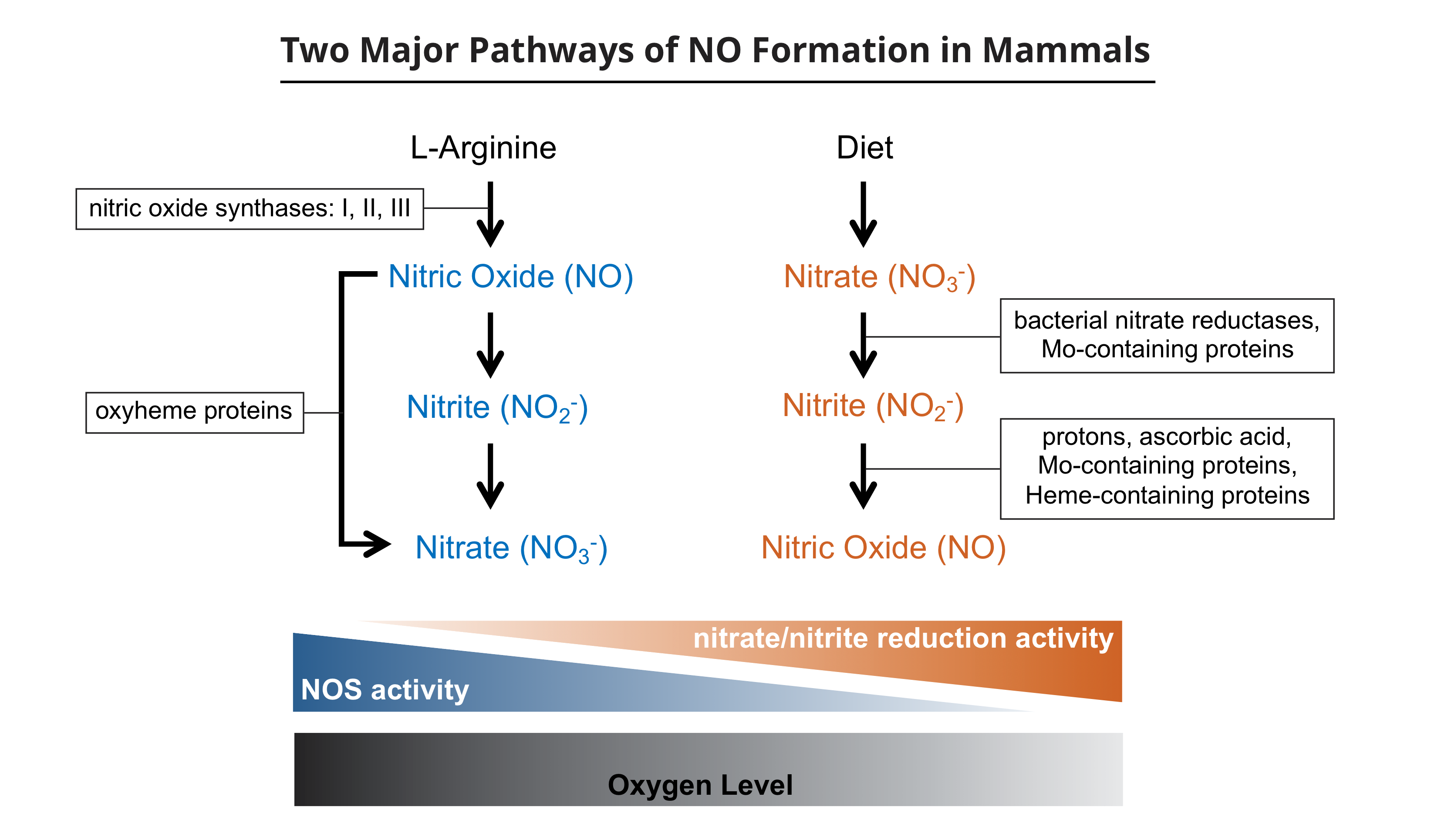
On the right is represented the more recently understood reductive pathways, leading to the formation of NO, which are more important under relative hypoxic conditions. These pathways include the actions of oral and gut bacteria but are also facilitated by various enzymes and other molecules in mammalian cells.
Abbreviation: NOS, nitric oxide synthase enzymes
Identifying human physiological processes involving Nitric Oxide
Our research programme in recent years has, in addition to such studies, focused on identifying human physiological processes which may be involved in producing NO by the reductive mechanisms as these may be affected by addition of nitrite or nitrate ions by diet or by other means for various pharmacological effects.
Briefly, we have found that platelet activation in rodents and people is inhibited by the effects of partially deoxygenated red blood cells in reducing blood nitrite ions to produce NO which inhibits several of the platelet activation pathways. Differences in blood clotting in arteries and veins, including the appearances of thrombi, may be due to how these processes are affected by the levels of oxygen saturation in the red cells interacting with the humoral biochemical processes initiated by thrombogenic stimuli.
We have also found that skeletal muscle, in several species including man, has very high levels of nitrate ions. Upon vigorous exercise this nitrate, which may come from diet or from NO synthesis by NOS I in muscle cells and then reaction with myoglobin, is reduced to nitrite and then NO. We believe this pathway contributes greatly to the very enhanced blood flow in muscles upon exercise. Indeed, it would appear that muscle may be the major reservoir for nitrate storage in the mammalian body.
Currently, we are also studying the formation and function of NO in animal and human eyes, in which reductive pathways beginning with nitrate ions may account for much of the NO which is important in retinal blood and intra-ocular fluid flows in the eye.
All of these studies have the potential for opening up new approaches to regulating NO levels in various tissues and organs for therapeutic goals.
Please note: This is a commercial profile