A group of academic experts from the U.S. and the UK share their views on effective regenerative medicine-based strategies against cancer
Among vertebrates, adult urodele amphibians retain the capability to regenerate complex structures such as limbs, tail, jaws, eyes and a variety of internal organs following an insult1. Similarly, but with a more limited potential, reptiles possess the capability to regrow a fraction of their tail dropped as a self-defence mechanism to elude a predator’s grasp. The strategies used by these highly regenerative organisms to restore organ function typically involve the crucial role of connective tissue-associated fibroblasts that undergo de-differentiation2.
The undifferentiated phenotype acquired by resident fibroblasts is the source of a complex embryonic structure called blastema, where the correct pattern for tissue growth is ensured by the positional identity of every single cell and by the continuous interplay between neighbouring cells3. This means that a precise recapitulation of specific embryonic developmental steps is required for successful tissue regeneration. Pro- and anti-inflammatory signals are timely induced following an insult, for instance, axolotl limb amputation and sustained throughout the process of regeneration, due to the transient presence of macrophages4.
This precise process is perpetuated until tissue restoration is completed and most interestingly, without incurring abnormalities or genetic aberrations. Rather, it has been observed that such a regeneration-permissive environment can reverse tumorigenicity by reprogramming tumour cells5. As an example, the administration of chemical carcinogens induces cancer in regeneration-incompetent tissues (i.e. the newt dorsal iris), but not in those capable of regeneration (i.e. the newt ventral iris)6. Specifically, despite a low frequency, malignant transformations can be observed together with the formation of invasive epithelial tumours, this phenomenon is generally followed by spontaneous remission as tumour cells are induced to reorganise into non-cancerous tissues7.
We thus hypothesise that the adult mammal’s susceptibility to cancer development has been determined by the loss of an advanced regenerative capability during the course of evolution, with the consequent lack of an associated control system. A better understanding of this evolutionary process can help conceive effective regenerative medicine-based strategies against cancer.
In adult mammals, only a rudimentary regenerative potential is evident in the form of quiescent cells, known as endogenous stem cells, because this feature is not sufficient to spontaneously regenerate a whole functional organ following an injury. On the other hand, we think that mammalian embryos keep the memory of the ancestors, as the controlled series of processes that resemble blastema formation described in amphibians are identified during embryonic tissue development as well. To state some common characteristics, embryos harbour an enormous number of undifferentiated cells, with a great proliferation rate, specific differentiative potential and a proper identity leading to morphogenesis (see Figure 1)8.
Similar to the blastemal environment of urodeles, the mammalian embryonic environment critically supports normal tissue development and precludes malignant transformations. In fact, besides the more efficient and frequent DNA repair mechanisms active in embryonic cells, other processes including apoptosis and senescence have also been reported as recurrent tumour suppressive systems acting to functionally remove cells at a risk of aberrant growth during embryogenesis9.
The above mentioned transient mechanisms, with characteristics that disappear at birth, have also been associated with the recruitment of macrophages for the clearance of suppressed cells10. The latter aspect is of particular interest because the presence of such inflammatory cells to the site of action is essential for the final outcome, whether during tissue regeneration, cancer progression, or morphogenesis.
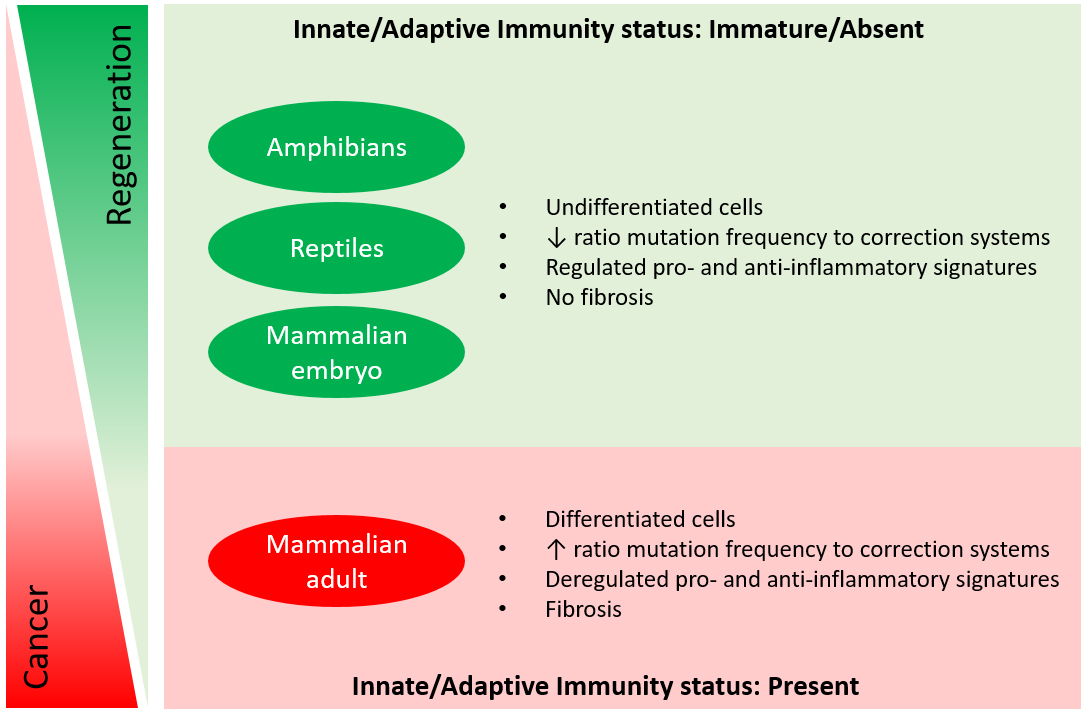
Like a wound that never heals, neoplastic transformation has been associated with persistent inflammation and abnormal macrophage differentiation11. The reactive species produced by these cells (i.e. reactive oxygen and nitrogen species) have been directly linked to an altered DNA repair, apoptosis and/or cell cycle checkpoint control12. As a consequence of an impaired wound healing process, chronic inflammation leads to fibrotic scar formation, whose role in tumorigenesis is still under debate. Some researchers consider fibrosis responsible for disruption of the anatomical polarity of a regenerating tissue and consequently for tumorigenesis following an injury, while others contemplate it as an alternative to cancer, thus protecting undifferentiated cells from uncontrolled proliferation that could represent a danger in case of chronic inflammation.
Based on these pieces of evidence, several approaches have been developed in the field of tissue engineering, which aim to reduce inflammation while avoiding scar formation and consequently to improve regeneration13-15. Our group recently demonstrated that the implantation of a finely functionalised collagen-based immunomodulatory material was capable of recruiting and modulating macrophage phenotype towards an anti-inflammatory status, therefore, recreating in mammals the pro-regenerative environment described in highly regenerative organisms16.
On the other hand, conventional tumour-associated macrophages displaying an anti-inflammatory phenotype are potent suppressors of anti-tumour immunity, whereas appropriately activated macrophages have been reported to lead to cancer-related inflammation and kill tumour cells17.
It becomes clear that within their dual role and multiple functions, macrophages hide the secret behind the uncontrolled tumorigenic condition frequently reported in adult mammals, as well as in the controlled cascade of events leading to embryogenesis. Surprisingly, embryos and highly regenerative organisms lack an established innate and adaptive immunity that is rather observed in animals with a more limited regenerative potential, including mammals, birds and reptiles18. The regenerating limb of newts suggests that similar to the role they play in embryogenesis and contrarily in tumorigenesis: macrophages are mainly required to mediate immunosurveillance and the clearance of senescent cells generated during blastema formation19 and to prevent the lysis of dedifferentiating cells induced by NK cells20.
In a nutshell, embryonal macrophages know how to create and support the microenvironment needed to guarantee tissue homeostasis and morphogenesis, while keeping it oncosuppressive. Understanding the interactions occurring at a microscopic level in the blastemal and embryonic tissues and keeping in mind the phylogenetically conserved properties between amphibians and mammals, we will be able to develop effective regenerative medicine-based strategies to fight cancer.
References
1 Stocum, D.L. Mechanisms of urodele limb regeneration. Regeneration 4, 159-200 (2017).
2 Odelberg, S.J. in Seminars in cell & developmental biology, Vol. 13 335-343 (Elsevier, 2002).
3 Brockes, J.P. Amphibian limb regeneration: rebuilding a complex structure. Science 276, 81-87 (1997).
4 Godwin, J.W., Pinto, A.R. & Rosenthal, N.A. Macrophages are required for adult salamander limb regeneration. Proceedings of the National Academy of Sciences 110, 9415-9420 (2013).
5 Tsonis, P. Effects of carcinogens on regenerating and non-regenerating limbs in amphibia. Anticancer research 3, 195-202 (1983).
6 Oviedo, N.J. & Beane, W.S. in Seminars in cell & developmental biology, Vol. 20 557-564 (Elsevier, 2009).
7 Fior, J. Salamander regeneration as a model for developing novel regenerative and anticancer therapies. Journal of Cancer 5, 715 (2014).
8 Blau, H.M. & Pomerantz, J.H. Re “evolutionary” regenerative medicine. Jama 305, 87-88 (2011).
9 Pawelec, G., Goldeck, D. & Derhovanessian, E. Inflammation, ageing and chronic disease. Current opinion in immunology 29, 23-28 (2014).
10 Storer, M. et al. Senescence is a developmental mechanism that contributes to embryonic growth and patterning. Cell 155, 1119-1130 (2013).
11 De Visser, K.E., Eichten, A. & Coussens, L.M. Paradoxical roles of the immune system during cancer development. Nature reviews cancer 6, 24 (2006).
12 Schäfer, M. & Werner, S. Cancer as an overhealing wound: an old hypothesis revisited. Nature reviews Molecular cell biology 9, 628 (2008).
13 Godwin, J.W., Pinto, A.R. & Rosenthal, N.A. in Seminars in cell & developmental biology, Vol. 61 71-79 (Elsevier, 2017).
14 Godwin, J.W. & Rosenthal, N. Scar-free wound healing and regeneration in amphibians: immunological influences on regenerative success. Differentiation 87, 66-75 (2014).
15 Corradetti, B. The immune response to implanted materials and devices. The Immune Response to Implanted Materials and Devices (2017).
16 Corradetti, B. et al. Immune tuning scaffold for the local induction of a pro-regenerative environment. Scientific reports 7, 17030 (2017).
17 Mantovani, A., Allavena, P., Sica, A. & Balkwill, F. Cancer-related inflammation. Nature 454, 436 (2008).
18 Mescher, A.L. Macrophages and fibroblasts during inflammation and tissue repair in models of organ regeneration. Regeneration 4, 39-53 (2017).
19 Yun, M.H., Davaapil, H. & Brockes, J.P. Recurrent turnover of senescent cells during regeneration of a complex structure. Elife 4, e05505 (2015).
20 Godwin, J., Kuraitis, D. & Rosenthal, N. Extracellular matrix considerations for scar-free repair and regeneration: insights from regenerative diversity among vertebrates. The international journal of biochemistry & cell biology 56, 47-55 (2014).
Please note: this is a commercial profile
Bruna Corradetti
Department of Nanomedicine,
Houston Methodist Research Institute
Centre for NanoHealth,
Swansea University Medical School
Prashant Dogra
Mathematics in Medicine Program,
Houston Methodist Research Institute
Mauro Ferrari
Department of Nanomedicine,
Houston Methodist Research Institute
Department of Medicine,
Weill Cornell Medical College
Vittorio Cristini
Mathematics in Medicine Program,
Houston Methodist Research Institute
Department of Imaging Physics,
University of Texas MD Anderson Cancer Center