Senior scientist Shima Kadkhodazadeh discusses adapting the optical properties of nanoparticles with high precision through alloying
Successful implementation of many technologies relies on synthesising materials with specific properties. In the case of nanoparticles, tailoring and fine-tuning their properties through precise control over their shape, size, crystal structure and composition is required. Alloying provides the appealing prospect of combining the desirable traits of different elements and using their relative composition to design new materials. In some cases, interesting synergetic characteristics and enhancements beyond those of the constituent elements can occur. For example, in catalysis, enhanced catalytic activity of bimetallic alloys in certain reactions have attracted much interest1. Another example is in plasmonic hydrogen sensing, where alloying Pd with other metals such as Au and Cu has been shown to result in improved sensitivity and accuracy of detection2–4. In plasmonic gas sensors, changes in the localised surface plasmons (LSPs) of metallic nanoparticles is used to detect the amount of gases present in the environment. This technique is particularly attractive for the detection of hydrogen. This is because H2 is a highly flammable gas and many conventional H2 detectors are electronic devices, which carry the risk of spark generation.
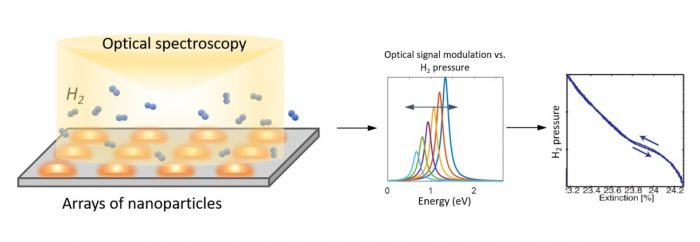
Given the exotic functionalities of many alloy nanoparticles, it is clear that their properties such as the crystal structure, band structure and permittivity function cannot be simply interpolated based on those of the elements they are composed of, or even assumed based on what is known of the alloys in bulk form. Therefore, it is highly important to characterise the crystallographic, electronic and optical properties of ‘nanoalloys’ experimentally, as well as develop new models to describe them. Recently, we have used scanning transmission electron microscopy (STEM) and electron energy-loss spectroscopy (EELS) to systematically study the LSPs and the permittivity functions of AuPd nanoparticles as a function of composition5. AuPd nanoalloys are of particular interest in plasmonic H2 sensing but their optical properties are not yet fully understood. The choice of STEM-EELS allows measurement of optical properties down to spatial 1 nm resolution, which is at least an order of magnitude better that what can be achieved with the best optical techniques.
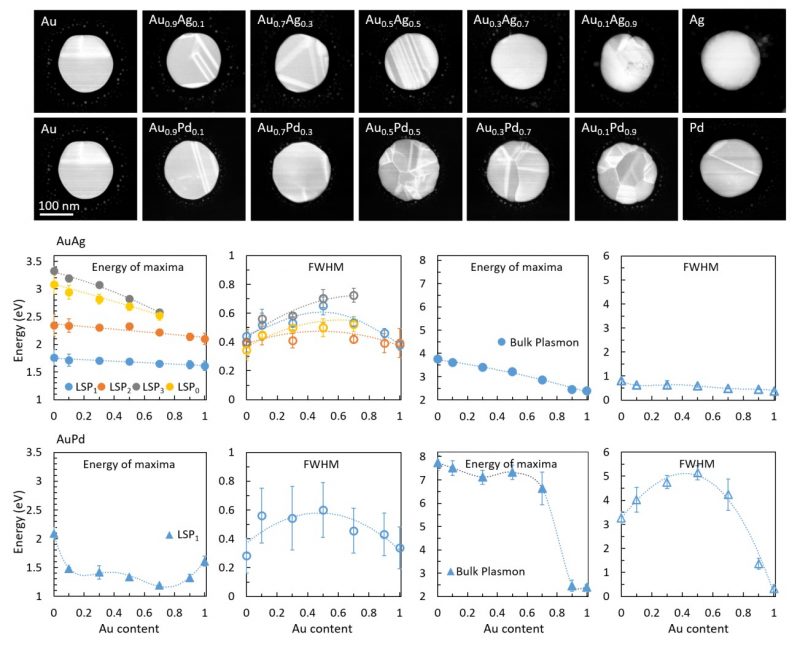
To benchmark the method, it was also applied to the more widely studied AuAg alloy nanoparticles, and good agreement was found between our STEM-EELS measurements and the previous experimental and theoretical studies of the permittivity function in these nanoalloys5. Our results indicate a stark difference between the behaviour of AuAg and AuPd alloys. While alloying Au with Ag results in relatively smooth changes in optical properties, AuPd alloys exhibit very non-linear optical characteristics as a function of composition. This is clearly seen when comparing the LSPs and the bulk plasmon behaviour in AuAg and AuPd nanoparticles vs. their Au content (Figure 2). The same trend is reflected in their permittivity functions (Figure 3) and originates from the changes occurring to the electronic bandstructure of these alloys with varying composition. Using our measurements, we could determine the bound electron contribution term, ε∞, in the Drude model of the permittivity function of AuAg and AuPd nanoalloys. This provides a simple but fundamental description of the optical response of AuPd alloys, required for accurately modelling, designing and optimising new and exciting optical devices.
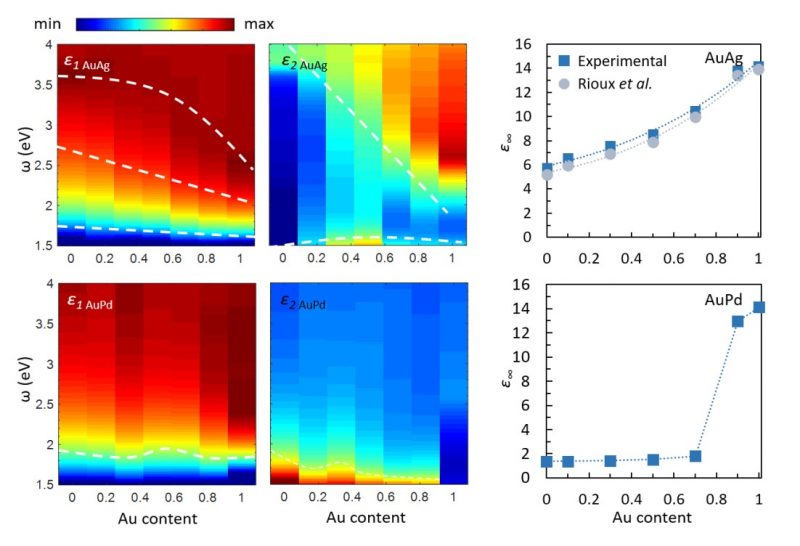
Senior Scientist Shima Kadkhodazadeh
DTU Nanolab
Technical University of Denmark
Tel: +45 4525 6494
shka@dtu.dk
References
(1) Liu, X.; Wang, D.; Li, Y. Synthesis and Catalytic Properties of Bimetallic Nanomaterials with Various Architectures. Nano Today 2012, 7, 448–466.
(2) Wadell, C.; Nugroho, F. A. A.; Lidström, E.; Iandolo, B.; Wagner, J. B.; Langhammer, C. Hysteresis-Free Nanoplasmonic Pd–Au Alloy Hydrogen Sensors. Nano Lett. 2015, 15, 3563–3570.
(3) Darmadi, I.; Nugroho, F. A. A.; Kadkhodazadeh, S.; Wagner, J. B.; Langhammer, C. Rationally Designed PdAuCu Ternary Alloy Nanoparticles for Intrinsically Deactivation-Resistant Ultrafast Plasmonic Hydrogen Sensing. ACS Sensors 2019, 4, 1424–1432.
(4) Nugroho, F. A. A.; Darmadi, I.; Cusinato, L.; Susarrey-Arce, A.; Schreuders, H.; Bannenberg, L. J.; da Silva Fanta, A. B.; Kadkhodazadeh, S.; Wagner, J. B.; Antosiewicz, T. J.; et al. Metal–Polymer Hybrid Nanomaterials for Plasmonic Ultrafast Hydrogen Detection. Nat. Mater. 2019, 18, 489–495.
(5) Kadkhodazadeh, S.; Nugroho, F. A. A.; Langhammer, C.; Beleggia, M.; Wagner, J. B. Optical Property–Composition Correlation in Noble Metal Alloy Nanoparticles Studied with EELS. ACS Photonics 2019, 6, 779–786.
(6) Rioux, D.; Vallières, S.; Besner, S.; Muñoz, P.; Mazur, E.; Meunier, M. An Analytic Model for the Dielectric Function of Au, Ag, and Their Alloys. Adv. Opt. Mater. 2014, 2, 176–182.