Researchers from Kyoto University Hospital and Preemptive Medicine and Lifestyle Related Disease Research Center, describe the future of vascular imaging technology, including how photoacoustic imaging targets tumour vessels in breast cancer
More than 70 diseases are attributed to excessive angiogenesis with inadequate function while angiogenesis plays an important physiological role in normal conditions, such as pregnancy, menstruation, and wound healing. By visualising the state of blood vessels within a disease, it may be possible to detect the disease at an early stage and monitor the effects of treatment.
Vascular imaging technology
We believe that such an imaging technology must have the following features.
First of all, it should be able to show blood vessels with high accuracy at a high resolution; secondly, it should be able to visualise the metabolic state of the blood; and finally, and especially important, it should be non-invasive.
Clinically feasible imaging modalities suitable for evaluating disease-related vessels include ultrasonography (with doppler, contrast-enhanced), angiography, computed tomography (CT), and magnetic resonance imaging (MRI). Conventional angiography is invasive and often paired with the intravascular treatment of the target lesion. Multidetector CT with contrast agent targeting vascular structure (CT Angiography) provides us with a detailed vascular network, however, it requires radiation exposure. MRI with a contrast agent may be an ideal imaging modality to evaluate disease-related angiogenesis without radiation exposure. Regarding cancer imaging with a contrast agent, selective visualisation of feeding arteries and drainage veins of tumours is currently available. Drainage veins were enhanced soon after feeding arteries are enhanced, suggesting the biology of tumour and tumour-associated vasculature such as increased intra-tumoral blood flow or intra-tumoral shunts. This short arterio-venous interval is characteristic of malignant tumours.
These conventional technologies have evolved to a great extent and are used in practice but somewhat invasive. We need to note that some patients cannot tolerate contrast media due to impaired kidney function or allergies, and radiation exposure is a concern for X-ray-based technologies. Thereby, we need a novel imaging system with a non-invasive approach.
Photoacoustic imaging technology
Photoacoustic imaging may be a breakthrough technology to solve these issues. It enables us to depict fine vessels without radiation exposure and the use of contrast media. Furthermore, photoacoustic imaging is also capable of visualising intravascular oxygen saturation in the 3D view as well.
In 1880, Dr Alexander Graham Bell first reported the photoacoustic effect. He showed that a sound generated through thermal expansion resulting from light energy could be detected with his stethoscope. This is called the photoacoustic effect. Unlike ultrasound, X-ray, nuclear medicine, CT and MRI, photoacoustic technology, which was discovered much earlier than any of these, has not reached practical use in medical settings yet.
The principle of photoacoustic imaging is as follows. A subject absorbs light energy, which generates a temperature rise and then results in the thermo-elastic expansion. That expansion generates sound emissions, and we can detect these sound signals emitted, and calculate the 3D location of the subject and visualise it on a computer screen.
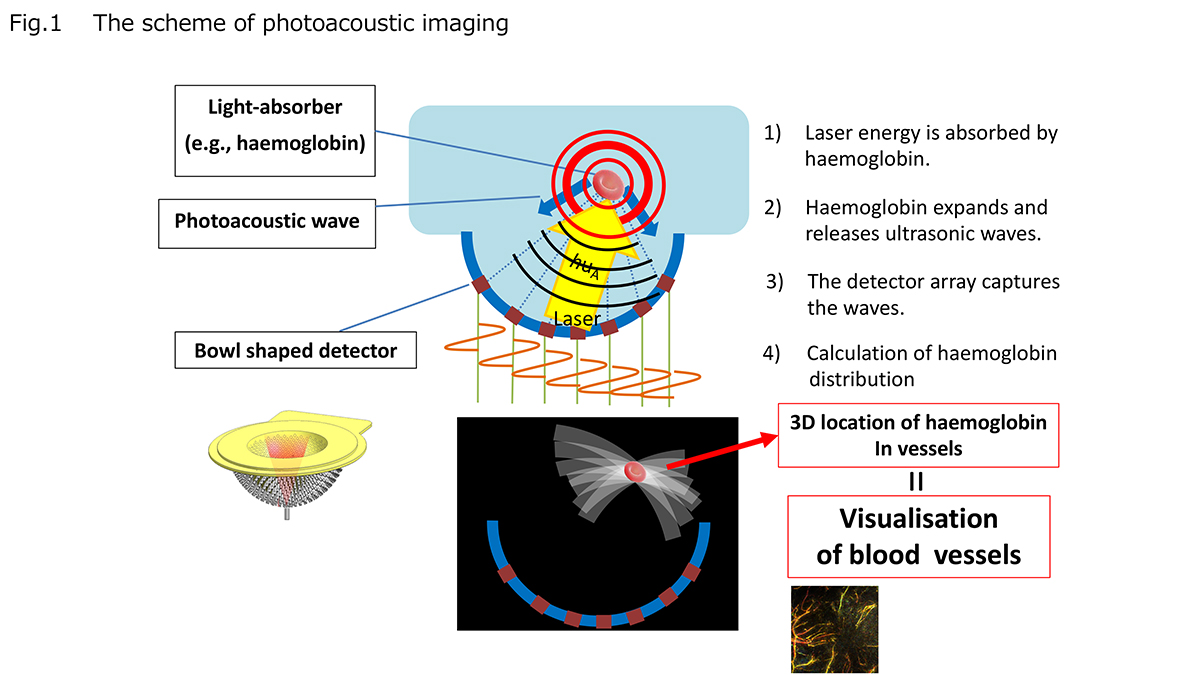
The haemoglobin, a major carrier of oxygen contained in red blood cells, absorbs the laser beam energy irradiated from the outside of the body. The thermo-elastic expansion of the haemoglobin elicited by the non-invasive laser beam releases ultrasonic waves, and these waves are captured at the detectors. The 3D position data of haemoglobin in vessels represent the blood vessel image (Fig.1).
Tumour-associated vessels are closely linked to the tumour growth activity and tumour-microenvironment. New vessel formation, or angiogenesis, is needed to respond to the increasing demand for oxygen and nutrients of growing tumours. Although normal and matured blood vessels are well organised, tumour-associated vessels are totally abnormal, such as dilated, tortuous, and leaky. Photoacoustic imaging is ideal for depicting such abnormalities of tumour-associated vessels.
Targeting tumour vessels in breast cancer
Our research group has focused on developing photoacoustic imaging equipment and system targeted at tumour vessels in breast cancer.
By matching the irradiated laser beam to the light absorption wavelength of haemoglobin, it is possible to visualise blood vessels as fine as approximately 200μm that cannot be detected by conventional imaging techniques, and also to calculate the oxygen saturation of the blood using the two wavelengths, 756nm and 797nm. On irradiation with 756nm light, oxygenated and deoxygenated haemoglobin have different absorption. In contrast, at 797nm wavelength, both absorption coefficients are the same. We call this calculated value the ‘S-factor’ saturation of oxygen. By irradiating the two wavelengths of the laser alternately, we eliminated the positional shifts and succeeded in acquiring high-resolution S-factor maps in human vessels in vivo (Fig.2).
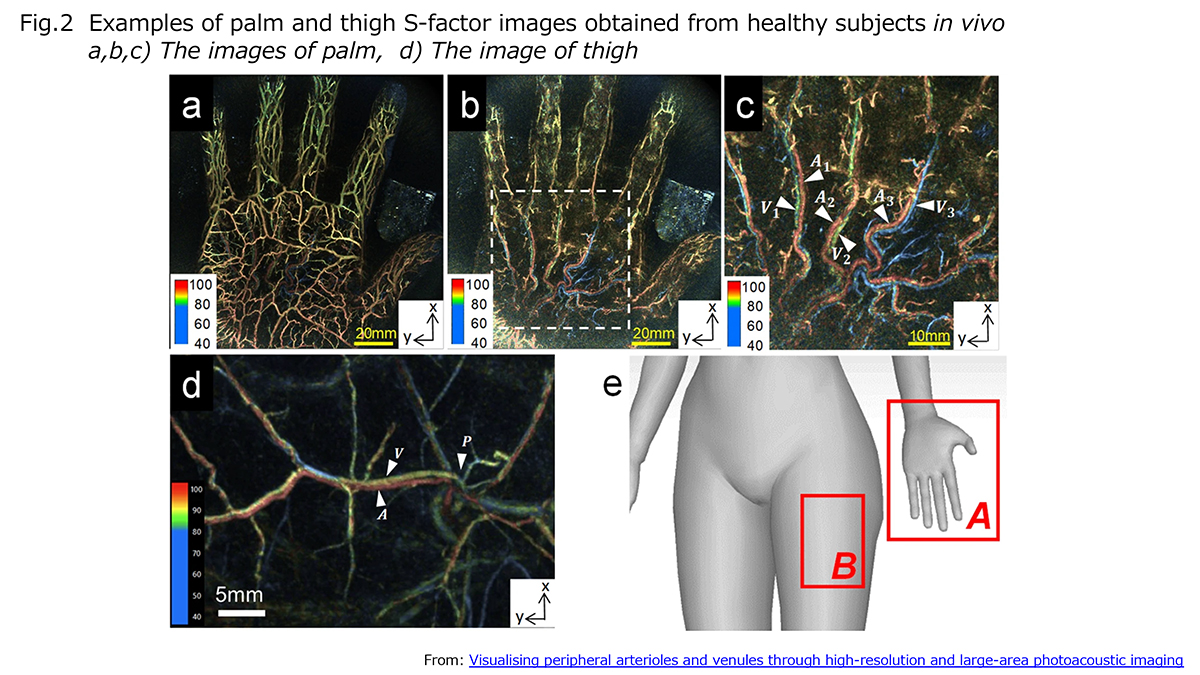
In oxygen saturation analyses in a clinical case of breast cancer, for example, we found low saturation signal spots emerging in the tumour tissue, and we observed changes in the vascular shape and S-factor status due to chemotherapy and molecular targeting treatments. These changes in the morphology and function of the vasculature, named ‘vascular normalization’, are expected to occur according to the treatment response and be observable by photoacoustic imaging.
A recently developed photoacoustic equipment visualised tumour vasculatures and S-factor oxygen status clearly, as shown in Fig.3 (triple-negative breast cancer). This photoacoustic imaging shows fine-bending blood vessels of the tumour. We understand clustered and irregular small arterioles and venules are developed by cancer in a systemic vascular network system.
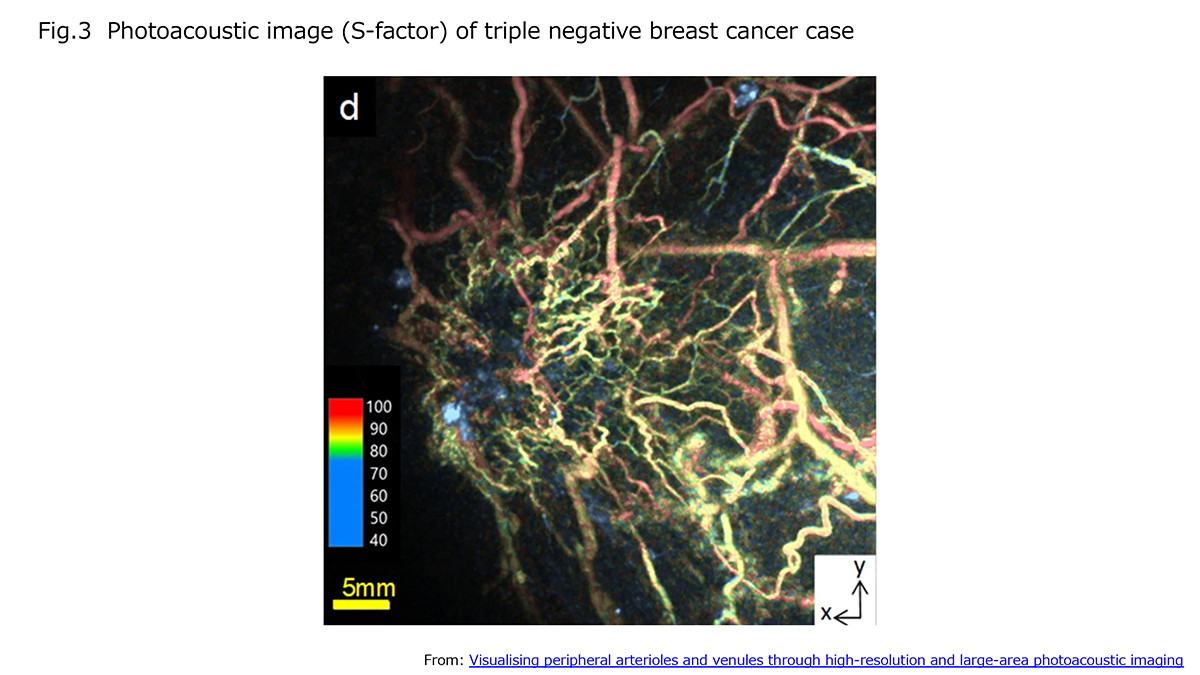
Photoacoustic imaging technique
In a sense, this photoacoustic imaging is a non-invasive modality that does not require radiation exposure or contrast medium. Our technique achieves a very high resolution in visualising tiny vessels in humans. Although we need to improve scanning depth further, this technique can depict fine small blood vessels and their oxygen saturation status that were previously impossible to visualise.
Photoacoustic imaging will make a variety of developments. Numbers of diseases are accompanied by abnormalities in blood vessels. Vascular abnormalities are engaged in not only cancers, but also cardiovascular diseases, thrombosis, diabetes and autoimmune diseases. Early detection and monitoring of these diseases by vascular imaging are crucial. In addition, S-factor imaging representing a metabolic status should be also helpful to understand the disease biology and treatment effects. Furthermore, vascular imaging is indispensable for plastic surgery, especially tissue transfer.
The future of imaging technology
In the future, we will be able to realise molecular imaging with photoacoustic technology. Potentially, a variety of probes are applicable in this system. For instance, remarkable imaging of lymphatic ducts and channels has already been achieved by using indocyanine green (ICG) as an imaging probe. In cancer treatment, we often use immune-oncological drugs nowadays. To understand what happens in tumour in the human body in vivo, visualisation of tumour microenvironment, not only vasculatures but also immune cell components are necessary. For this purpose, the photoacoustic imaging system would be helpful.
The photoacoustic imaging technology will contribute widely to the diagnosis of disease. The coupling of morphological imaging of vasculature and functional imaging of oxygen provides a new paradigm in the imaging field. It is warranted to study the development of practical equipment, the development of probes for photoacoustic imaging, and the integration of AI for diagnosis.
References
Onishi N et al. Ultrafast dynamic contrast-enhanced MRI of the breast using compressed sensing: breast cancer diagnosis based on separate visualization of breast arteries and veins. J Magn Reson Imaging. 2018;47:97-104.
Honda M et al. New parameters of ultrafast dynamic contrast-enhanced breast MRI using compressed sensing. J Magn Reson Imaging. 2020;51:164-174.
Matsumoto Y, Toi M. Women’s Imaging 2020 Breast Imaging Vol.15 Innervision, 35, 8, 48-51.
Toi M et al. Visualization of tumour-related blood vessels in the human breast by the photoacoustic imaging system with a hemispherical detector array. Scientific reports, 2017;7:41970.
Matsumoto Y et al. Visualising peripheral arterioles and venules through high-resolution and large-area photoacoustic imaging. Scientific reports, 2018, 14930.
Nagae K et al. Real-time 3D Photoacoustic Visualization System with a Wide Field of View for Imaging Human Limbs F1000Res. 2018 Nov 19;7:1813.
Itaru T et al. Photoacoustic Tomography Shows the Branching Pattern of Anterolateral Thigh Perforators In Vivo. Plast Reconstr Surg. 2018 May;141(5):1288-1292.
Kajita H et al. Photoacoustic lymphangiography J Surg Oncol. 2020;121:48-50.
Described by Masakazu Toi1, Yoshiaki Matsumoto1,2, and Masako Kataoka3
1: Breast Surgery, Graduate School of Medicine, Kyoto University
2: Preemptive Medicine and Lifestyle Related Disease Research Center, Kyoto University Hospital
3: Diagnostic Imaging and Nuclear Medicine, Graduate School of Medicine, Kyoto University
This research was funded by The ImPACT Programme (programme manager Takayuki Yagi), Cabinet Office, Japan
Please note: This is a commercial profile