Senior researcher at Center for Electron Nanoscopy at DTU CEN, Takeshi Kasama reveals the institution’s expertise around transmission electron microscopy
Transmission electron microscopy (TEM) involves the use of high-energy electrons that have passed through a thin specimen to record images, diffraction patterns or spectroscopic information. Many different TEM techniques have been developed over the years into highly sophisticated methodologies that have found widespread application across many scientific disciplines.
Since the TEM has an incomparable capability to provide structural and chemical information over a range of length scales down to atomic dimensions, it has developed into an essential tool for researchers who are interested in understanding the properties of nanostructured materials and in manipulating their behavior. Besides the conventional TEM techniques, TEM offers a unique opportunity for imaging ‘functionalities’ and ‘material property interactions’ at the nanoscale. Electron holography allows magnetic and electrostatic fields in nanostructured materials to be recorded quantitatively at a high spatial resolution down to ~5 nm, while Lorentz microscopy can be used to image their dynamical changes from large areas of interest1.
Figure 1 demonstrates a magnetic induction map of a ferromagnetic CoPd nanoparticle encapsulated in a multi-walled carbon nanotube recorded using electron holography, which may recall a magnetic flux pattern formed using a bar magnet and iron filing.
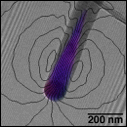
One of the scientifically attractive systems is the switching of material properties by the interplay between magnetism, electricity and elasticity (e.g., multiferroicity). Magnetite, which is a naturally occurring multiferroic mineral, undergoes a first-order phase transition below ~125 K, known as the Verwey transition, from a cubic structure to a closely related monoclinic structure. The loss of the symmetry results in the formation of ferroelastic transformation twins, which consequentially produce spontaneous strains.
Electron holography and Lorentz microscopy were used to understand local interactions of ferrimagnetic domain walls with the ferroelastic twin walls2. Figure 2 shows that magnetic domain walls appear reproducibly at the same positions after applying external fields to the specimen by partial excitation of the microscope objective lens, suggesting that the positions of the magnetic domain walls are defined strictly by underlying nanoscale twin domain walls.
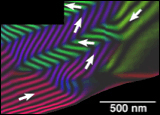
The magnetic imaging techniques have also been also applied to geoscience-related subjects, such as how rocks record magnetic fields and retain them over a geological timescale. In many cases, geological samples contain different magnetic minerals and/or magnetically different phases even though they are the same mineral. TEM can obtain local magnetic information from individual magnetic phases and correlate the magnetic characteristics directly with other physical, chemical or geometrical properties.
Electron holography was used to elucidate magnetic mineral’s ability to retain the past magnetic field that existed when our solar system was formed3. Nanometre-scaled tetrataenite was magnetically stable enough for maintaining the acquired magnetic fields and had recorded the magnetic information along the axis of time as if it was a magnetic tape recorder3, 4.
What is coming next? At DTU-Cen, we are especially interested in observing ‘functionalities’ and ‘dynamic behavior’ in actual reaction environments. Electron holography and Lorentz microscopy in gas atmospheres using environmental TEM or liquids would open a new avenue to nanoscale magnetic research that had not been considered until recently. The combination of the magnetic imaging and environmental TEM allows us to study how redox modifies magnetic structures along with the material phase and composition in desired atmospheric environments5.
The validity of in-situ liquid-cell electron holography to the detection of magnetic and electrostatic fields has been proven in a few groups including DTU-Cen. This technique would provide opportunities to investigate magnetic interactions between nanoparticles in liquid and magnetisation evolutions during crystal growth and dissolution. Thus, the magnetic imaging would become a more attractive tool for studying local magnetic properties in actual environments and device’s working conditions and could be used in broader research fields, such as material science, geoscience, environmental science and medical and biological applications.
References
1 T. Kasama, R.E. Dunin-Borkowski, M. Beleggia, in: Holography – Different Fields of Application, edited by F.A. Monroy Ramírez, InTech, pp. 53-80 (2011).
2 T. Kasama, R.J. Harrison, N.S. Church, M. Nagao, J.M. Feinberg, R.E. Dunin-Borkowski, Phase Transit. 86, 67 (2013).
3 J.F.J. Bryson, N.S. Church, T. Kasama, R.J. Harrison, Earth Planet. Sci. Lett. 388, 237 (2014).
4 J.F.J. Bryson, C.I.O. Nichols, J. Herrero-Albillos, F. Kronast, T. Kasama, H. Alimadadi, G. van der Laan, F. Nimmo, R.J. Harrison, Nature 517, 472 (2015).
5 T. Almeida, T. Kasama, A.R. Muxworthy, W. Williams, L. Nagy, T.W. Hansen, P.D. Brown, R.E. Dunin-Borkowski, Nature Commun. 5, 5154 (2014).
Please note: this is a commercial profile
Takeshi Kasama
Senior researcher
DTU CEN – Center for Electron Nanoscopy
Tel: +45 4525 6475