Here, M. Danner & R. M. Winglee* describe the viability of microbial sampling within impact lander craters in extraplanetary ice, including the possibility of life beyond our planet
With the possibility for life outside of our planet being an important and intriguing question, the search for easier mechanisms to reach potentially life-sustaining habitats is a crucial endeavour for the space sciences. Though traditional landing systems for off-world exploration like the NASA Mars rovers are highly adept, the systems are highly complex and prone to failure when put to extended distances such as Jupiter or Saturn. Due to the potential pitfalls of complex landing mechanisms, more direct systems are being designed and tested.
Landing systems
Landing systems such as the Europa Kinetic Ice Penetrator’s (EKIP) “cryo-braking” system have been proposed as an efficient and straightforward way to land on airless extra-terrestrial bodies, such as comets or icy moons Europa and Enceladus. These icy bodies are prime targets for astrobiological research due to the occurrence of water ice and possible liquid water in the subsurface. The EKIP system (Fig. 1) works primarily through a hollow penetrator impacting the surface of the icy body and creating a columnated ejecta plume of ice and detritus. A secondary system, the lander, follows behind and interacts with the ejecta, transferring momentum from the icy debris to the lander, much like the effect of a parachute in an atmosphere. Once the lander is safely on the surface of the icy body, sampling for microbial life of both the plume and the newly disturbed surface (the crater) can commence.
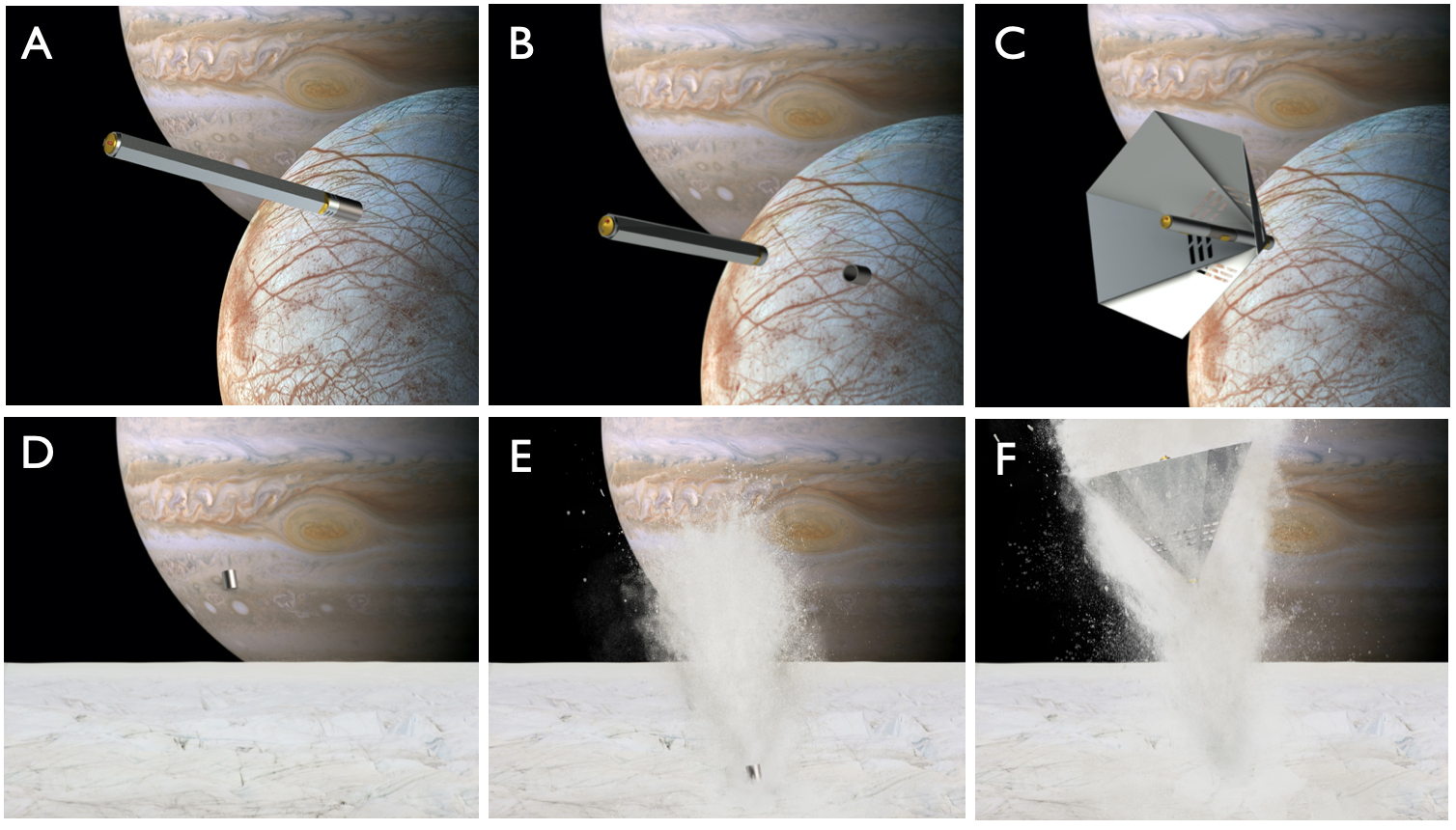
The ejecta turnover of the surface actually benefits the sampling of possible microbes due to excavation of deeper material. Radiation from the solar wind and Jupiter’s torus would destroy any evidence of microbial life within roughly the first meter of the surface, so any sample must be from below that threshold. However, with such a violent method of descension, it is imperative to determine whether the landing mechanism destroys all evidence of possible microbial life.
To determine the viability of this landing system for missions involving microbes, one meter diameter containers of ice were frozen to -20 C in 10cm layers. Once frozen to 70 cm depth, a 20 cm diameter saline ice cylinder embedded with the microbe Planococcus halocryophilus OR1 (PHOR1), a so-called microbial plug, was placed into the centre of the main ice container. This particular extremophilic microbe was chosen for its ability to survive extremely low temperatures, as well as being intolerant of freshwater.
Additionally, the PHOR1 microbe has a simplistic cellular structure that is easier to identify in microscopic analysis. This was done to avoid contamination issues arising from the experimental setup. Due to the inability of the microbe to occur naturally in lab water, any occurrence is noted as part of the embedded microbes from the experiment. The microbial plug was then surrounded by freshwater ice to create a smooth surface. Once frozen through, the entire cylinder is frozen to -30 C, and set to be impacted with miniaturised versions of the EKIP penetrator as tested in previous studies.
The ice was impacted at 2.2 km/s, with ejecta being collected with a blast shield directly opposite the crater, as well as a sterilised tarp that collected larger detritus. Additional samples were collected from within the intact crater within the microbial plug. Samples were moved frozen until analysis was possible.
Analysing samples
Samples were analysed using the dye LIVE/DEAD, which utilises two fluorescent dyes, red and green, of differing sizes. If a cell wall is intact and unharmed, inferring the microbe is alive, the red dye molecule is too large to enter the cell, while the green dye can stain the inner cellular material, resulting in the living microbe being dyed green. If the cell wall is damaged, the larger red dye enters the cell and shows the dead microbial waste as dyed red. The benefit of this approach is that even damaged or dying microbes are able to be identified, instead of relying purely on living sample.
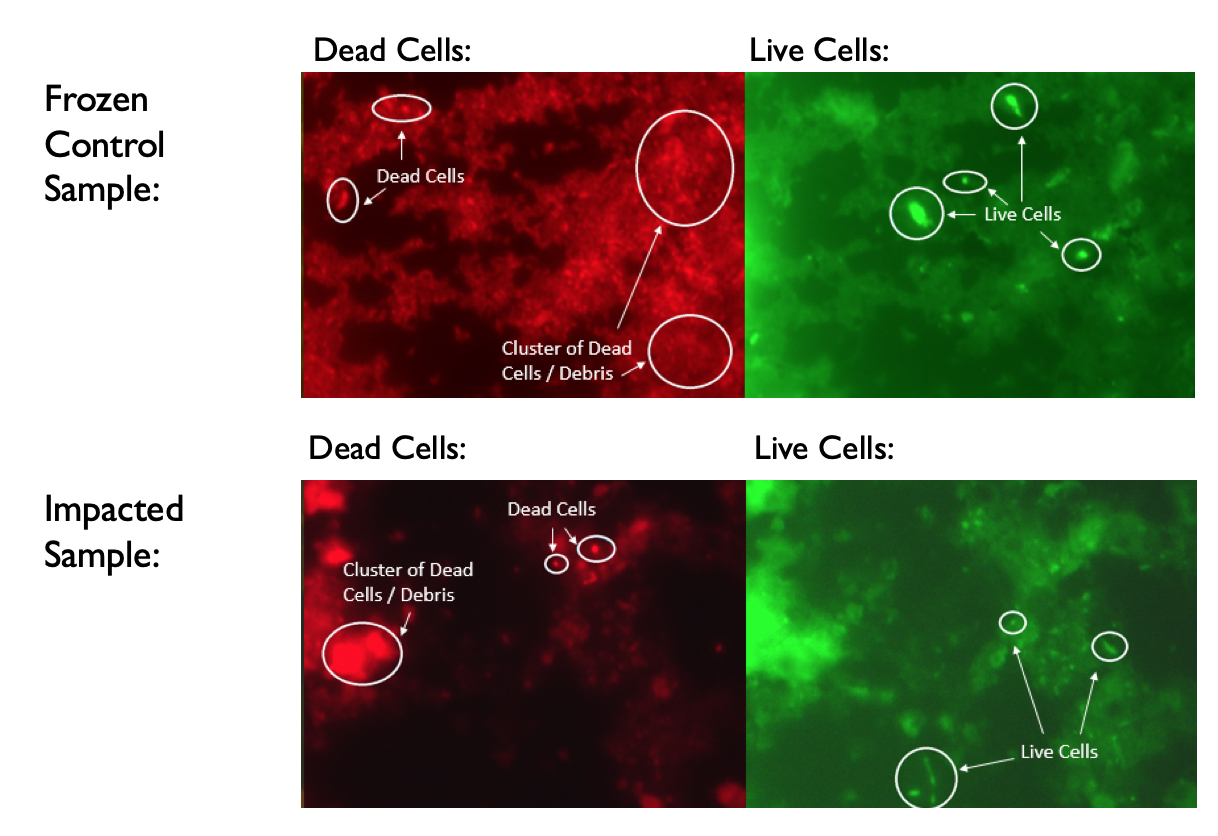
As shown in Figure 2, merely freezing the microbe in with our methodology caused large amounts of die-off. With that being said, PHOR1 microbes were still viable before impact. In the impacted sample, yet more die-off occurred, but yet again, noticeable amounts of the microbe are still discernable.
With the visibility of living PHOR1 within the impacted sample, it can loosely be determined that the EKIP impact landing mechanism is a viable option for missions to icy bodies. Possible living microbes could potentially be found using motion-sensing microscopes aboard the lander. Additionally, the presence of even the damaged microbial debris shown in red would be measurable by DNA sequencing of melted sample. This means that even if potential microbes are killed by the landing mechanism, evidence of their existence is still discoverable.
*R.M. Winglee passed away Dec. 2020
References
Winglee, R. M. et. al., “Cryo-braking using penetrators for enhanced capabilities for the potential landing of payloads on icy solar system objects”. Acta Astronautica, Volume 144, p. 136-146.
Robinson, Tessa, Robert M. Winglee, and Carl Knowlen. Europa Kinetic Ice Penetrator System for Hyper Velocity Instrument Deposition. Thesis. University of Washington, 2017. Print.
Please note: This is a commercial profile